Search Results
544 items found for ""
- 22 Inspiring Examples of Mechanical CAD Designs for Real-World Applications
Why CAD Revolutionized Mechanical Engineering Computer-aided design (CAD) software has transformed mechanical engineering over the past several decades. While engineers once relied solely on paper blueprints and manual drafting techniques, CAD enabled the rapid digitization and automation of design work. No other technology has had a greater impact on mechanical engineering in recent history. This article will explore the evolution of CAD and its expanding role in the field. We’ll cover topics including: The transition from paper drafting to digital design The capabilities of modern CAD programs Real-world examples and applications The benefits CAD delivers for engineers Modeling methods like parametric vs direct The future of CAD in mechanical engineering By the end, you’ll understand exactly why CAD became an indispensable tool for creating everything from consumer gadgets to advanced manufacturing equipment. The stunning designs made possible by CAD showcase how this software revolutionized mechanical engineering. A Brief History of CAD Computer-aided design (CAD) has transformed the field of mechanical engineering. Prior to the introduction of CAD software in the 1960s, mechanical designs were created manually using paper and drafting tools. This traditional drafting method involved meticulous attention to detail, long lead times, and difficulty modifying designs. The origins of CAD can be traced back to proprietary electronics design systems developed in the 1950s and 1960s. However, it wasn't until the 1970s that CAD software became more mainstream for mechanical design with the release of early 2D drafting programs like CADAM and CATIA. These introduced digital drawing boards and allowed basic 2D drafting and dimensioning. Major innovations came in the 1980s and 1990s with the development of 3D CAD modeling. Parametric 3D CAD programs like Pro/ENGINEER and SolidWorks revolutionized mechanical design by enabling the creation of complex 3D models. Parts and assemblies could now be modeled, rendered, and analyzed on the computer. Today CAD is ubiquitous in mechanical engineering. Continued improvements in 3D modeling, multidisciplinary analysis, and specialized CAD applications have made computer-aided design an indispensable tool for modern mechanical engineering. The manual drafting methods of the past have become obsolete. Benefits of CAD Computer-aided design (CAD) software has revolutionized the field of mechanical engineering and changed the very nature of the design process. CAD provides a number of key benefits that have dramatically improved mechanical design: Speed CAD software enables engineers to design parts and assemblies much faster than traditional manual drafting methods. Design iterations can be turned around rapidly, accelerating the design process. Changes are also easy to make with CAD models. Accuracy CAD allows for an unprecedented level of design precision. Components can be modeled, measured, and toleranced to within thousandths or millionths of an inch. This accuracy carries through to manufacturing as CAD models are used for CNC programming. Reduced Costs By enabling faster and more precise design work, CAD software reduces project costs significantly. Less time is spent drafting, and manufacturing errors are minimized. CAD also allows for design optimization, reducing material waste. Design Complexity CAD removes the limitations of manual drafting, enabling engineers to design geometries and assemblies too complex to draw by hand. CAD allows for the precise design of intricate components critical to many industries. Analysis and Optimization CAD software facilitates engineering analysis like FEA, CFD, and more. Digital prototypes can be simulated and optimized before physical prototypes are built. This prevents costly redesigns and improves quality. Overall, CAD empowers engineers to design higher quality products in less time and at lower costs than traditional methods. It paved the way for today's complex mechanical devices across industries like aerospace, automotive, and medical. CAD Software Options CAD software comes in many forms, each with their own strengths and ideal applications. Here are some of the most common CAD platforms used in mechanical engineering: SolidWorks SolidWorks is one of the most popular 3D CAD modeling tools used by mechanical engineers. It utilizes a parametric feature-based approach to modeling. SolidWorks is known for its user-friendly interface and extensive design, simulation, and documentation capabilities. It's commonly used for complex part and assembly modeling across industries like automotive, aerospace, and industrial machinery. AutoCAD AutoCAD by Autodesk is a premier CAD software for 2D drafting and detailing. It allows users to create flat designs and blueprints. AutoCAD lacks built-in 3D modeling capabilities but can be upgraded to a 3D version. It remains a standards-based desktop application for 2D drafting tasks like architecture and engineering drawings. CATIA CATIA by Dassault Systèmes is a high-end CAD package used for 3D product design. It offers advanced surface and solid modeling for designing complex aerospace, automotive, and industrial products. CATIA is known for exceptional knowledge reuse, collaboration, and PLM integration. Creo Formerly known as Pro/ENGINEER, Creo Parametric by PTC is another leader in 3D parametric CAD software. It provides a full spectrum of 3D CAD modeling and product design capabilities. Creo is highly customizable and extensible, making it well-suited for large enterprises. Inventor Inventor by Autodesk is 3D CAD modeling software made for mechanical designers and engineers. It utilizes a parametric, direct edit solid modeling approach. Inventor offers dynamic simulation, stress analysis, and tooling design capabilities. It integrates well with the Autodesk ecosystem. CAD Modeling Methods CAD software provides different modeling techniques to create 3D models and 2D drawings. The two most common methods are: Parametric Modeling Parametric modeling is based on creating models with parameters and relationships between features. The dimensions and constraints drive the geometry, so if you change a parameter, the entire model updates accordingly. Benefits of parametric modeling include: Easy to modify designs by changing parameters Features related through parent-child relationships Design intent is captured in the feature history Constraints help make valid models Integrates well with analysis tools Parametric modeling is most commonly used in mechanical engineering design. Popular parametric CAD packages include SolidWorks, CATIA, Creo, Inventor and NX. Direct Modeling In direct modeling, you directly manipulate the 3D geometry without having to worry about parent/child relationships or feature history. It focuses on the explicit geometry. Advantages of direct modeling: Flexible for quick design iterations Easier to import or repair geometries Intuitive push/pull editing of models No need to recreate feature history Good for complex organic shapes Direct modeling is incorporated into many CAD programs along with parametric tools. Some CAD platforms like Fusion 360 use a combination of both methods. Other modeling techniques like surface modeling, curve/wireframe modeling, and solid modeling may also be incorporated into CAD software. The modeling method depends on the specific engineering design workflow and application. Mechanical Engineering Applications Mechanical CAD has transformed design across mechanical engineering disciplines, enabling more optimized, economical, and innovative solutions. From the largest aircraft to the smallest precision instruments, CAD empowers engineers to bring their visions to life. Aerospace The aerospace industry relies extensively on CAD for every aspect of aircraft design. CAD models are used to design fuselages, wings, control surfaces, landing gear, and every other component. Engineers can test flight characteristics, structural integrity, aerodynamics, and manufacturability in CAD before any physical prototyping. Leading aerospace companies use CAD to design commercial airliners, fighter jets, helicopters, satellites, rockets, and more. Automotive The automotive industry was an early adopter of CAD, and car makers now develop entire vehicles digitally. CAD is used to design and optimize engine and drivetrain components, suspensions, vehicle body panels, interiors, frames, and more. Engineers can test aerodynamics, crashworthiness, ergonomics, manufacturability, and assemble entire vehicle CAD models. CAD enables automakers to quickly iterate designs to create better performing, safer, more fuel-efficient cars. Industrial Machinery Industrial machinery like CNC machines, robots, conveyor systems, and heavy equipment are all designed in CAD. It allows engineers to create complex mechanical systems and components like hydraulic systems, transmissions, motors, and compressors. CAD is invaluable for designing large equipment that would be difficult or impossible to prototype physically at early design stages. Companies rely on CAD to design machinery optimized for performance, reliability and manufacturability. Medical Devices The medical industry relies on CAD software for the precise design of implants, prosthetics, surgical tools, imaging equipment, and other devices. CAD allows for complex geometries, miniaturization, and customized patient-specific solutions. Engineers can ensure devices interface properly with anatomy and validate strength, longevity, and manufacturability in CAD before prototyping. CAD enables advanced medical solutions that radically improve patient outcomes. Innovative Examples Mechanical CAD allows engineers to create complex designs that would have been incredibly challenging or impossible with traditional drafting methods. Here are some standout real-world examples of innovative mechanical engineering designs made possible thanks to CAD. 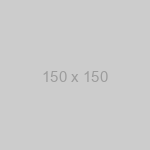 Electric Vehicle Powertrain This CAD model shows the complex powertrain system of an electric vehicle. The parametric 3D CAD environment enabled engineers to precisely design the electric motor, gearbox, axles, cooling system, and structural components to fit together seamlessly. Simulations also optimized the torque and power output. 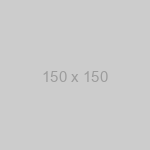 Bionic Prosthetic Hand Using CAD, engineers could mimic the anatomy of the human hand to design an advanced prosthetic hand with articulating fingers. The CAD model was used to 3D print prototypes and assemble the intricate components of the bionic hand. 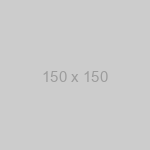 Jet Turbine Engine The many intricate components of a jet engine would be nearly impossible to design without 3D CAD modeling. Every part must perfectly fit together while handling incredible temperatures, stresses, and rotational speeds. CAD enables designers to visualize, simulate, and validate these life-critical aircraft systems. 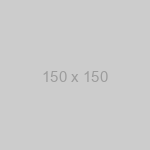 Laparoscopic Surgery Tool Doctors rely on complex tools designed using CAD for minimally invasive surgery. This CAD model was used to craft the precise components and linkages in the instrument for laparoscopic abdominal procedures. CAD helps maximize performance while allowing the device to fit through a tiny incision. 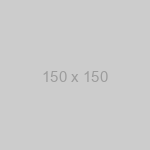 Consumer Appliance Enclosure Using CAD, consumer product designers can create ergonomic, aesthetically pleasing enclosures and housings. This CAD model shows the outer case for a kitchen appliance. CAD allows iterations of the smooth, sculpted geometry while maintaining proper clearances for internal components. 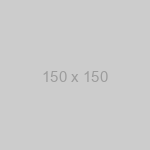 8-Speed Automotive Transmission The complex assembly of gears, shafts, and bearings in a modern 8-speed automatic transmission is developed entirely in CAD before any physical prototypes are built. CAD models inform each precisely engineered component and enables the system to be simulated under load. Let me know if you would like me to expand on any of these examples or add additional ones! Impact on Industry CAD has fundamentally transformed mechanical engineering and product design across nearly every industry. By enabling faster and more optimized workflows, CAD has helped accelerate product development cycles and allow for more complex designs. Whereas in the past, creating manufacturing designs was an analog process done by hand on paper, CAD has fully digitized the process. Engineers can now rapidly iterate through digital prototypes and simulations to refine products. This iterative process means mistakes and flaws can be caught and corrected early on through digital simulation and analysis. With CAD, far more complex designs can be rendered and tested virtually before physical prototypes are built. Creating intricate products with thousands of custom parts would simply be infeasible without digital modeling. CAD allows every nut, bolt, and component to be designed in coordination. By frontloading design validation in the digital realm, manufacturers can slash the time and costs spent on physical prototyping. Bringing products to market faster provides a significant competitive advantage. CAD enables engineers to respond quickly to changing customer needs and emerging use cases. The precision of CAD also creates possibilities for designing highly optimized components and systems. With CAD, mechanical engineers gain new abilities to simulate and test products under different scenarios, fine-tune specifications, and maximize performance objectives. The result is higher quality products that make the most of materials and manufacturing methods. In summary, CAD has been transformational for mechanical engineering across industries including automotive, aerospace, machinery, robotics, and consumer products. It has enabled faster development cycles and more complex, optimized designs that were simply not feasible using analog drafting techniques. CAD software has redefined manufacturing and will continue introducing new possibilities for product innovation. Future of CAD CAD software is continuously evolving to leverage new technologies like artificial intelligence, virtual reality, generative design, and more. Here are some exciting innovations on the horizon for CAD: Artificial Intelligence and Machine Learning AI and machine learning are being integrated into CAD platforms to automate and streamline workflows. For example, CAD programs can now automatically generate 3D models from 2D sketches, instantly convert hand-drawn sketches to precise CAD geometry, and even suggest design improvements to engineers. As AI continues improving, CAD will become even more intuitive and autonomous. Cloud-Based CAD Cloud technology enables real-time collaboration on CAD models from any location. Engineers can simultaneously work on a design, with changes syncing seamlessly across the team. Cloud-based CAD also provides easy mobile access and limitless computing power for analyzing large assemblies. Expect cloud platforms to dominate as they provide flexibility and accessibility. Generative Design Generative design uses algorithms to explore the full design space and produce countless iterations meeting requirements. Engineers define goals like weight, strength, and cost - then let the software optimize the form. This automated trial-and-error discovers high-performing designs unimaginable to humans. CAD will integrate generative technology to boost innovation. Virtual and Augmented Reality VR and AR have huge potential for visualizing CAD models at true-to-life scale before manufacturing. Design reviews, ergonomic evaluations, and assembly simulations can be done immersively in virtual reality. Augmented reality allows overlaying holographic CAD data onto real objects for analysis. As VR/AR grows, it will transform CAD workflows. Additive Manufacturing Also known as 3D printing, additive manufacturing technology is advancing rapidly. CAD models can be directly fabricated without additional tooling. Engineers can optimize designs for 3D printing, incorporating freeform geometry unproducible by other methods. Seamless integration of CAD and 3D printing will enable greater innovation and customization. In summary, CAD is poised for major innovations driven by emerging technologies. As CAD software leverages AI, VR, generative algorithms and additive manufacturing, it will enable engineers to design, optimize and produce mechanical creations previously unimaginable. The future of CAD in mechanical engineering is filled with exciting potential. Conclusion Mechanical CAD software has transformed the engineering design process. By enabling complex 3D models to be quickly created, modified, simulated, and shared digitally, CAD provides immense value over traditional paper drafting techniques. As outlined in this post, the key benefits of CAD for mechanical engineering include: Faster and more precise designs through computer automation Ability to create, analyze and optimize very complex components and assemblies Lower costs from reduced errors and digitally sharing/storing designs Improved quality and functionality of products through simulation Easy collaboration and design reviews with remote team members CAD has enabled mechanical engineers to develop incredible innovations across industries like aerospace, automotive, robotics, and more. Looking to the future, CAD will continue advancing through AI/ML integration and new capabilities like generative design. While CAD software requires an initial investment of time to learn, it pays exponential dividends for mechanical engineers by enhancing creativity, productivity and efficiency in developing cutting-edge products. This post aimed to provide both an overview and examples of CAD's transformative impact. The power of digital design is clear, and CAD will only grow more capable and essential for next-generation mechanical engineering.
- 16 Strategies for Collaborative CAD Design Projects in Mechanical Engineering Teams
Establish Clear Guidelines and Expectations Successful collaboration starts with establishing clear guidelines and expectations upfront. This involves clearly defining team member roles, setting realistic deadlines and milestones, and creating a shared vision. Define Team Roles and Responsibilities Appoint a project manager to oversee the entire project timeline, resources, and team. Identify a lead engineering designer to make final decisions on the design. Assign team members to specific subsystems and components. Be clear about ownership and responsibilities. Have frequent check-ins to realign if any role ambiguities emerge. Set Realistic Deadlines and Milestones Create a detailed project plan with key milestones for concept reviews, design reviews, prototyping, testing, etc. Break down larger milestones into smaller tasks with actionable deadlines. Build in buffer time for inevitable delays and rework. Reconfirm deadlines after each milestone to keep the project on track. Create a Shared Vision Hold a kickoff meeting to align on the end goal and key requirements. Document decisions. Develop renderings and specs that represent the shared vision of the final product. Use this as a reference to keep team priorities aligned throughout the project. Leverage Cloud-Based CAD Platforms for Real-Time Collaboration Cloud-based CAD platforms enable real-time collaboration on design projects. Rather than emailing files back and forth, cloud platforms allow multiple team members to access and edit the same CAD models simultaneously. This facilitates rapid iteration and co-creation. Popular cloud-based CAD options include Onshape and Autodesk Fusion 360. These provide features like unlimited version history and branching, allowing teams to efficiently track changes from different designers. Version control gives teams visibility into who changed what, when. This makes it easy to understand design evolution and rollback modifications if needed. With cloud CAD, designers don't have to wait for teammates to finish their work before accessing the latest files. This improves workflow and allows for true concurrent engineering. Team members can also leave comments on models, creating discussion threads around specific design features. Overall, leveraging cloud-based CAD platforms is crucial for facilitating collaboration in modern engineering teams. The accessibility, transparency and flexibility provided gives designers the tools needed to work together effectively. Encourage Open Communication and Feedback Regular meetings and check-ins are essential for collaborative CAD projects. The team should hold regular status updates, design reviews, and Q&A sessions. This gives everyone a chance to get on the same page, provide feedback, and ask clarifying questions. Team members should also feel comfortable providing timely, constructive feedback to one another. Feedback helps improve designs and prevents costly mistakes. Team leads can encourage feedback by creating a safe, supportive environment. Lastly, no question should go unasked. Even if it seems trivial, asking questions prevents assumptions which can lead to problems. Team members should never hesitate to speak up if they need clarification or more context. The end goal is creating the best product, not looking foolish in front of coworkers. Implement Best Practices Following industry best practices and standards is crucial for streamlining collaboration in CAD design teams. This establishes consistency and organization across the entire design process. Here are some key best practices to implement: Standardize Part Naming Conventions Agree on a part numbering system upfront. Some options are alphanumeric codes or sequential numbers. Make part names descriptive yet concise. Avoid overly vague or ambiguous names. Indicate the material and process in the name, if applicable. Use consistent abbreviations and syntax for the naming convention. Organize Files and Folders Create a logical folder structure to categorize parts, assemblies, drawings etc. Name folders clearly based on product, subsystem or design version. Establish permissions for who can access or modify each folder. Update Master Files Regularly Maintain a single master file with the latest design version. Have a process for routinely updating this master file as changes occur. Ensure only authorized users can modify the master to avoid version conflicts. Archive older design iterations in a separate folder as backups. Following these and other best practices will help CAD teams stay coordinated, streamline handoffs, quickly locate files, reduce errors, and maintain organized design data. Standards empower consistent collaboration. Provide Ongoing Training and Support Effective training is crucial for maximizing productivity and collaboration on CAD design teams. Teams should start by providing comprehensive onboarding and training on the chosen CAD platform and project management software. Hands-on exercises, videos, guides and in-person walkthroughs can help team members quickly get up to speed. It's also important to offer refresher courses or advanced training periodically. As team members gain experience, additional training can help reinforce best practices and optimize workflows. Members can also be cross-trained on different aspects of the CAD software over time. Finally, assign mentors and coaches to provide guidance and answer questions, especially for new team members. Experienced users can share insights on the platform capabilities, shortcuts, customization and more. Allow time for mentees to shadow their mentors and learn directly from observation. Proper mentorship ensures skills are passed on and team members continue to develop their abilities. With ongoing CAD and project management training, teams can avoid frustrations from knowledge gaps. Team members will feel empowered to use the tools effectively and collaborate smoothly on designs. Divide Work Systematically Based on Parts, Assemblies and Subsystems When collaborating on complex CAD design projects, it's critical to systematically divide up the work based on parts, assemblies and subsystems. Rather than haphazardly assigning tasks, teams should take a strategic approach. First, clearly define all the parts, assemblies and subsystems that make up the overall product. Then systematically assign responsibility for designing each component. Having team members focus on particular sections prevents duplicate work and avoids gaps. Allow for some intentional overlap between team members. Collaboration doesn't happen in silos, so make sure assignments have cross-functional elements. For example, two designers can work on connecting components that will interface, meeting regularly to align. For exceptionally large and intricate CAD projects, use the "divide and conquer" strategy. Break the design into smaller segments and have sub-teams tackle each piece in parallel. Just ensure proper coordination and clear hand-offs as the sub-assemblies come together into the final product. The key is to proactively carve up the work, rather than reactively working on whatever seems most urgent. This prevents tendencies to silo or over-focus on pet projects. Systematic division of labor reduces bottlenecks, keeps everyone optimized, and delivers seamless collaboration. Enable Effective Decision Making Effective decision making is critical on collaborative CAD projects to align the team and move the design process forward. Teams should decide when it makes sense to use individual vs group decision making. For decisions that impact the full team, group decision making is preferred. This allows multiple perspectives to be gathered and increases buy-in once a decision is made. There are several proven strategies for group decision making: Voting: Each team member casts a vote, and the option with the most votes wins. This is quick and straightforward, but majority rule isn't always the ideal outcome. Consensus: The team discusses all options and aims to reach a decision everyone actively supports or at least consents to. This ensures broader agreement but can be time-consuming to achieve full consensus. Compromise: Neither side gets exactly what they want, but everyone gets some of what they want. This can help find middle ground when consensus can't be reached. It’s critical to have a clear process for resolving conflicts and disagreements that arise during collaborative CAD projects. Consider designating a neutral party to mediate if needed. Allow both sides to explain their reasoning, then aim for a solution that satisfies everyone involved as much as possible. With the right decision making strategies in place, teams can effectively align around design choices and minimize conflict. This enables smoother collaboration and faster progress on complex CAD projects. Manage Data Flow and Version Control To ensure seamless collaboration, mechanical engineering teams need to establish systems to manage data flow and version control of CAD design files. This involves setting up protocols for sharing updated files, enabling "check-in" and "check-out" systems, and carefully merging changes from different team members. One effective strategy is to maintain a central cloud-based folder that acts as the single source of truth for the latest CAD design files. Team members can upload and download updated versions from this folder. Permissions can be set to restrict editing access as needed. Version control is critical when multiple engineers are working on the same assemblies and parts. A check-in/check-out system should be used, where team members "check-out" a file before making changes, and "check-in" the modified file when done. This ensures two people don't overwrite the same file. Changes from different engineers will need to be integrated through careful merging. It's important to regularly synchronize and incorporate changes from across the team into the master CAD models. Communication is key - noting the types of revisions made and any potential conflicts. With thoughtful data management protocols, mechanical teams can streamline collaboration and ensure everyone is working from the most up-to-date CAD files. This avoids duplication of work and catastrophic losses of progress. Allow for Design Flexibility and Changes When collaborating on CAD design projects, it's important to build in flexibility and remain open to design changes during the process. The initial concepts and proposals will likely evolve as the project progresses, based on testing results, design challenges that emerge, feedback from team members, and new requirements or considerations that come up. Rather than sticking rigidly to the original design, teams should expect and embrace changes. Having an inflexible mindset will only lead to frustration when reality diverts from the plan. By contrast, welcoming modifications empowers the team to continuously refine and optimize the design. It's wise to plan ahead for potential rework by building in time buffers. Depending on the complexity of the project, teams may want to allocate extra time for redesigning and modifying components or even starting over on certain parts. This prevents rushed or sloppy work when last-minute changes are needed. Mechanical engineers collaborating on CAD should remain open-minded, even if changes are proposed in the final stages. While significant revisions late in the process can be disruptive, they occasionally produce breakthrough innovations that greatly improve upon the initial design. Weighing the trade-offs, costs and benefits will determine whether a change is worthwhile at a given point. But outright dismissing suggestions simply because of bad timing risks missing out on major enhancements. By embracing uncertainty and change, teams can maximize creativity. The collaborative process works best with some fluidity, rather than narrowly sticking to pre-determined solutions. While the original vision provides direction, organic growth and evolution is usually needed to achieve an optimal design. Review and Approve Designs at Key Milestones Reviewing and approving designs at major milestones is critical for successful collaboration on CAD projects. This allows the team to align, provide feedback, and ensure the design meets requirements before moving forward. The key milestones to review are: Initial Concepts and Proposals: The team should review initial design concepts, sketches, and proposals before beginning detailed CAD work. This allows for alignment on the overall design direction and can prevent wasted efforts from starting detailed work too early. Have the team provide constructive feedback at this stage. Detailed Designs: Once detailed CAD design work begins, the team should review and officially approve these designs before any prototypes are built or parts are ordered. This prevents costly errors from parts being made to incorrect specifications. Final Designs Before Release: The last milestone is a final review and sign-off on designs before they are formally released from the CAD system and shared with manufacturing. This ensures all team members are aligned that the design meets requirements and is truly ready for production. Following this structured review and approval process at three key milestones allows mechanical engineering teams to collaborate smoothly on CAD projects. It enables alignment while allowing flexibility for needed design changes. Reviews also provide critical checkpoints to catch errors before they lead to wasted time and money down the road.
- 9 Ways to Optimize Your CAD Models for 3D Printing in Mechanical Engineering
1. Simplify Geometries When designing parts for 3D printing, start by simplifying the geometry as much as possible. Complex models with lots of intricate details can be difficult and time-consuming to print. Instead, focus on the key features the part needs to function properly. Reduce the number of small features like holes, ribs, or text. These fussy details are difficult for the printer to recreate accurately and can lead to print failures. Stick to the essentials needed for the part to work. Use basic geometric shapes like squares, rectangles and cylinders rather than complex freeform curves and surfaces. Printers find it easier to smoothly fill in layers for fundamental shapes. Avoid adding any tiny precision features smaller than around 0.4mm. Most desktop 3D printers cannot print details below this threshold, resulting in rough or inaccurate surfaces. By removing unnecessary elements and optimizing your CAD model geometry early on, you can dramatically reduce print time and chances of print failure. Printers will have an easier time recreating the simplified part layer by layer. 2. Design Parts as Assemblies When 3D printing complex mechanical parts, it is often better to split the design into sub-assemblies that can be printed separately and then joined together. This allows you to optimize the orientation and print settings for each component. It also avoids common printing issues caused by large, intricate models. Design your assemblies in CAD software by modeling connectors between the separate components. This allows the parts to precisely fit together after printing. Some best practices include: Split Into Sub-Assemblies - Identify sections of your model that can be divided into logical sub-assemblies. Keep components under a certain max size based on your printer build volume. Design Proper Connectors - Add connectors like pins, joints, and fasteners so the sub-assemblies can be mechanically assembled after printing. Design connectors that interlock cleanly without needing supports. Account for Tolerances - Allow for print tolerance of around 0.1-0.3mm when modeling connectors and assembly clearances. 3D printing is not always dimensionally accurate, so design assemblies with appropriate tolerances. By designing your complex mechanical parts as assemblies, you can optimize the orientation and print settings for each component. This will result in higher quality 3D printed parts that assemble properly. 3. Optimize Wall Thicknesses When designing parts for 3D printing, one of the most important considerations is setting the optimal wall thickness. The wall thickness will impact the part's strength, material usage, print time and quality. Follow your printer manufacturer's guidelines for minimum wall thickness based on the nozzle size. Most FDM printers require walls to be at least 2-3x the nozzle diameter. For SLA printers, the minimum is around 0.7-1mm. Going below these thresholds risks print failures and quality issues. Account for the strength characteristics and printing behavior of your chosen material. For example, flexible materials can be printed thinner than rigid plastics. Parts under high stress need thicker walls for sufficient strength. Reference material datasheets for recommendations. Balance strength requirements with print time. Thinner walls print faster but compromise durability. Medium thicknesses around 2mm provide a good balance. Critical load-bearing features may need 4-5mm. Solid blocks maximally strengthen parts but require very long print times. For complex organic shapes, variable thickness walls that follow form can save material and weight while maintaining strength. Use thicker fortifications only where needed. Whenever possible, orient the part to minimize thin cross-sectional walls, which are more prone to breakage. By optimizing your model's wall thicknesses for the printer, materials, and application, you can achieve faster print times while still guaranteeing the required structural integrity. Print tests will help dial in the ideal values. 4. Orient Parts Properly One of the most important aspects of optimizing mechanical CAD models for 3D printing is properly orienting the part on the print bed. The orientation can have significant effects on print time, material use, and part strength. When orienting parts, the goal should be to minimize overhangs and reduce the need for support structures. Overhangs are sections of the print where layers have nothing underneath them. This commonly happens when printing angles greater than 45 degrees. In these situations, supports are required during printing to prevent drooping or collapse. Supports add print time, use extra material, and can be tricky to remove. To avoid overhangs, orient the part so the majority of surfaces are printed flat against the build plate or only have shallow angles. Some things to consider: Place high-detail surfaces face down. This takes advantage of the highest resolution on the first layers. Rotate the part so the shortest dimension is on the Z axis. This minimizes layer height and reduces print time. Orient parts vertically for columns or cylinders to avoid printing overhangs around the circumference. Proper orientation also optimizes part strength based on the expected load direction. For example, parts will be strongest when layers are perpendicular to the primary force. Consider analysis like FEA to determine the optimal orientation. Lastly, orientation affects cooling. Parts should be oriented to allow even cooling of layers. Avoid situations where one section cools quickly while another overhang section is still being printed. The uneven cooling can cause warping or delamination. Taking the time to thoughtfully orient your CAD parts will pay off with faster prints, less material use, and optimal mechanical properties. Analysing overhangs, supports, strength, and cooling will lead to a smarter orientation. 5. Design Efficient Supports One of the keys to optimizing mechanical CAD models for 3D printing is designing efficient support structures. Support structures are crucial for successfully printing overhangs and complex geometries. However, supports also increase print time, material use, and post-processing work. When designing supports, follow these best practices: Adhere to Overhang Rules: Most slicers use a 45 degree rule - any overhangs greater than 45 degrees will require supports. Some flexible materials allow for more overhang before needing supports. Analyze overhangs and only use supports where truly needed. Use Optimized Support Patterns: Choose an optimal support pattern based on your part's geometry. Dense supports or a lattice pattern works well for large overhangs. Tree supports help reduce material use and removal time. Position Supports for Easy Removal: Situate supports so they can be easily removed after printing. Avoid overhanging supports as much as possible. Place supports uniformly to minimize risks of part warping. Efficient support design takes some trial and error. But following these tips will help mechanical engineers 3D print complex parts faster, with less material waste and post-processing work. Analyze overhangs, utilize optimized patterns, and position for easy support removal. 6. Choose Suitable Infill Infill refers to the structure inside the walls of a 3D printed object. It's usually a lattice or grid pattern that provides internal support. Choosing the right infill settings can have a major impact on both the strength and print time of your parts. The key is finding the ideal balance between these two factors based on your specific mechanical design needs. When optimizing infill, start by considering the load conditions your part will experience. Parts that need to withstand high stresses or forces will require a higher infill percentage, such as 40-60%. This adds strength but also increases print times. For parts that don't experience high loads, a lower infill around 20% is often suitable. This greatly reduces print time while still providing adequate strength for the application. You can also adapt the infill pattern or type to suit the design. For example, gyroid and cubic infills offer a good compromise between print speed and strength. Linear patterns print fastest but can compromise strength on the horizontal plane. Finally, consider how you orient the infill structure. Aligning it with the forces applied to your part can maximize strength along those load paths. Rotating the infill 45° or 90° from the main axes may provide benefits depending on the design. Experiment with different infill settings when optimizing your CAD models. Pay attention to how infill orientation, pattern, and density impact the print time versus strength trade-off. Finding the right balance will allow you to 3D print functional mechanical parts faster. 7. Refine Problematic Areas When 3D printing mechanical parts, certain geometries can cause issues during the printing process. Areas like sharp corners and thin regions tend to have lower strength and may warp or deform. Optimizing these areas before printing can improve part quality. One technique is to add fillets and radii to sharp corners. The smoother curvature distributes stresses over a larger volume and reduces the likelihood of cracking. For functional parts, balance larger fillets for strength with smaller ones for fit and clearance. Trapped powder inside hollow sections can also lead to defects. Adding small escape holes allows powder removal before and after printing. Position the holes strategically to minimize visible surfaces. Thin regions like ribs and bosses can droop or fail in the middle layers. Adding a rib structure reinforces thin features while minimizing material use. Orient ribs vertically for better strength than horizontal ribs. Focus on ribbing walls, bosses, pads, and any thin or undersupported areas. Taking the time to refine tricky geometries before printing can prevent wasted time and material. Simple changes like fillets, escape holes, and ribs make parts more robust and optimized for 3D printing. Evaluate your model and address weak points proactively. 8. Perform Design Validation Before sending your CAD model to print, it's critical to validate the design by previewing it in your slicer software and test printing iteratively. This helps catch any potential issues that could ruin a print or waste time and materials. When previewing the layer view in your slicer software, look for the following: Overhangs - Any areas without support can droop or sag if the angle is too sharp. Add supports in these areas. Bridges - Sections that span a gap without underlying support. Ensure bridged areas are not too long. Thin walls - Very thin vertical or horizontal walls may not print properly. Consider making them thicker. Clearance - Check that moving parts have enough clearance to move freely after printing. Test print your model and inspect carefully: Monitor the first layers to ensure good adhesion and confirm your raft, brim or skirt is working properly. Check for signs of sagging, warping or layer shifting and adjust if needed. Iterate by tweaking printer settings, orientation, infill, supports and model design issues. Validate your model fully before committing to a final print. Good design validation prevents wasted time and materials. 9. Optimize Printer Settings Getting the most efficient and high-quality 3D prints starts with optimizing your printer settings. Here are some best practices: Calibrate Your Machine Before printing anything, it's crucial to fully calibrate your 3D printer. Calibration ensures components are aligned, leveled, and operating smoothly. Go through your printer's full calibration sequence, checking the guide rods, belts, hotend, and print bed. For the best dimensional accuracy, precisely calibrate the steps/mm on each axis. Use Quality Filament Not all filaments are made equal. Quality filament from reputable suppliers is recommended for mechanical parts. The diameter tolerance and consistency of the filament matters, as inconsistencies can lead to clogged nozzles or weak prints. Opt for filaments optimized for mechanical applications. Dial In Print Settings Take the time to properly dial in all your print settings, including temperature, print speeds, retraction, and cooling. Most slicers provide presets, but fine tuning for your specific printer and part will produce better results. Run test prints to optimize settings like temperature, print speed, layer height, infill, etc. Monitor the First Layers The first few layers set the foundation for the entire print. Closely monitor the first layers; if they don't adhere well or gaps appear, stop the print and re-level the bed. Adjust the z-offset and try again until you achieve a solid first layer. Choose the Right Nozzle Larger nozzles and layer heights speed up prints significantly but reduce detail and precision. For mechanical parts, a smaller nozzle like 0.2 or 0.4mm is recommended for precise details, tolerances, and a good surface finish. Only go larger if timeline is critical but precision is not. By properly calibrating your machine, using quality filament, optimizing print settings, monitoring initial layers, and selecting the ideal nozzle size, you'll achieve faster, higher-quality 3D prints. Dialed-in printer settings let your optimized mechanical CAD models shine. 10. Post-Process Parts Post-processing involves additional steps taken after a part is 3D printed to improve the final product. Properly post-processing parts can enhance the quality, appearance, and tolerances of 3D printed mechanical components. Common post-processing techniques for 3D printed parts include: Remove Supports Support structures help hold up overhanging sections while printing, but need to be detached after the print is finished. Support material can often be removed by hand, using pliers or flush cutters to clip away supports. For difficult to reach areas, an Exacto knife or rotary tool can help slice away excess material. Be sure to remove all remnants of support material so the final surface is smooth. Sand/Finish Surfaces The layer-by-layer printing process can leave visible striations and a rough texture on the exterior walls of a part. Sanding helps smooth down the stepped layers into a more uniform surface. Start with a coarse grit sandpaper (100-150) to rapidly remove bigger imperfections, then gradually step down to finer grits (up to 2000) for polishing. Alternative finishing techniques include bead blasting or vapor smoothing. Improve Tolerances/Cosmetics Post-processing can also improve the dimensional accuracy of printed parts. Sanding, machining, or tumbling can help bring critical mating surfaces and holes to more precise tolerances. Techniques like priming, painting, and plating can also give parts a more refined, finished look and feel. Properly leveraging post-processing techniques helps 3D printed mechanical parts meet functional requirements while achieving a smooth, polished appearance. Parts can be sanded, machined, coated, and finished to improve quality and cosmetics after initial printing.
- 18 Resources for Learning Mechanical CAD from Beginner to Expert Level
Introduction to Mechanical CAD Design Mechanical CAD (computer-aided design) software allows engineers and designers to create accurate 2D drawings and 3D models of mechanical parts and assemblies. It is an essential tool for any mechanical engineering or manufacturing related field. The goal of this guide is to provide a thorough overview of learning resources to master mechanical CAD, from beginner to expert level. Mechanical CAD software includes programs like AutoCAD, AutoCAD Mechanical, Inventor, Creo/Pro-Engineer, SolidWorks, CATIA, NX Unigraphics and Solid Edge. These tools allow users to draft 2D geometry, create 3D part and assembly models, generate detailed engineering drawings, simulate motion or stress analysis, and document product specifications. Most platforms also include capabilities for sheet metal, weldments, surfacing, CAM and more. Learning mechanical CAD requires understanding the software, interface, settings, drafting tools, modeling techniques, and industry practices. A combination of methods like online courses, blogs, tutorials, instructor-led training and hands-on practice is key. This guide will provide a roadmap of the top learning resources across all the key mechanical CAD knowledge areas. With structured and guided learning, anyone can master mechanical CAD design from beginner to professional levels. The resources shared will introduce the fundamentals, build key skills, and help you become an expert CAD designer or drafter. Getting Started with AutoCAD AutoCAD is a complex software with many features and capabilities, but you don't need to learn everything at once when you're just starting out. Here's an overview of the key concepts and tools you need to begin creating 2D drawings in AutoCAD. The AutoCAD Interface When you first open AutoCAD, you will see the main application window which contains the ribbon interface at the top with various tabs like Home, Insert, Annotate, etc. These tabs contain related tools and commands to create and edit your drawings. The drawing area is blank at first, that's where you'll create your drawing by adding lines, circles, text and other objects. On the left you'll find the tool palettes which provide quick access to commonly used blocks and tools. At the bottom is the command line, this allows you to type commands and values instead of using the ribbon. The status bar runs along the bottom displaying the current properties like the coordinates of your cursor. Creating Objects To begin a drawing you need to create lines and shapes. The common tools for this are located on the Home tab. Some basics: Line: Draw straight line segments. Circle: Draw circles by specifying radius or diameter. Arc: Draw curved arcs. Rectangle: Draw rectangles by specifying opposite corners. Polyline: Create connected straight and curved segments. You can enter exact values to place objects precisely using the dynamic input at the cursor. Layers Layers allow you to organize different types of objects. For example, you may have separate layers for walls, doors, text. To create a new layer, type `LAYER` or use the Layer Properties Manager. Then you can assign objects to different layers as you draw. Dimensions Adding dimensions is crucial for conveying the precise size of objects in CAD drawings. Use the Dimension tool on the Annotate tab to add horizontal, vertical, aligned and angular dimensions. Select the objects to dimension, then choose the dimension type and place the dimension line. You can fine tune the appearance and precision of the dimensions. This covers the very basics of starting a drawing in AutoCAD. Next we'll look at more advanced 2D drafting and detailing capabilities. 2D Drawing and Drafting Orthographic projection is essential for any 2D CAD drawing. This involves creating different views of an object - front, top, side etc. - that accurately represent the object's dimensions. When creating these views, it's important to follow orthographic projection rules to get the correct perspective and proportions. Section views are useful for showing internal features and details that aren't visible in regular orthographic views. To create a section view, you draw cutting lines to indicate where you want to cut the object, then use hatching, shading or other techniques to indicate the cut. Aligning section views properly with orthographic views is key. Annotations like dimensions, notes, symbols, and callouts are critical for conveying design intent and manufacturing specifications. Dimensions should be placed appropriately, formatted correctly, and use the proper dimensioning standards. Notes and callouts must clearly indicate what they refer to. Creating blocks for common symbols and annotations helps streamline the detailing process. Applying annotation styles helps maintain consistency and automatically update annotations if your standards change. Drafting tools like object snaps and polar tracking aid in precisely placing detailling geometry. Using layers organizes object types and allows controlling visibility. Proper detailing principles and CAD techniques are key for 2D documentation quality. 3D Modeling One of the key aspects of becoming proficient in AutoCAD is learning 3D modeling techniques to create and edit 3D objects. While 2D drafting is essential, 3D modeling allows you to design for real-world objects and environments. There are several important 3D modeling concepts and tools to learn in AutoCAD: Extruding and Revolving Extruding and revolving 2D objects are two of the most basic ways to create 3D forms in AutoCAD. The EXTRUDE tool allows you to select a 2D object like a line or polygon and extrude it into a 3D object by specifying a height. The REVOLVE tool lets you revolve or rotate a 2D object around an axis to create a 3D object. Mastering extruding and revolving is key to constructing basic 3D forms. Boolean Operations Boolean operations like UNION, SUBTRACT, and INTERSECT allow you to combine and manipulate 3D objects. The UNION tool merges two 3D objects into one unified object. SUBTRACT allows you to subtract one object from another. INTERSECT lets you create an object from the overlapping volume where two objects intersect. Boolean operations are critical for creating complex 3D models by combining and altering basic 3D forms. Rendering Rendering generates lifelike visualizations of 3D models by applying realistic lighting, materials, backgrounds, and effects. AutoCAD's built-in rendering engine allows you to create stunning rendered images directly from your 3D models. You can control lighting, materials, and backgrounds for photorealistic renderings. Rendering is important for visualizing and presenting 3D designs with real-world context and appearances. With these core 3D modeling tools and concepts, you'll be equipped to take your AutoCAD skills to the next level by designing, visualizing, and presenting 3D models. Hands-on practice with extruding, revolving, Boolean operations, and rendering will build expertise for tackling both simple and complex 3D projects in AutoCAD. Piping and P&ID Design AutoCAD provides dedicated tools for piping and process plant design. Piping Tools and Setup The AutoCAD Plant 3D toolset includes Pipe Network tools to layout and route piping systems with fittings, connectors, valves and supports. You can select pipe specs like material, diameter and standard from built-in catalogs. Pipe supports can be inserted and moved along the pipe runs according to code requirements. The Pipe Edit tool allows you to insert or move points on your pipe networks for optimal routing. Creating Schematics Process & Instrumentation Diagrams (P&IDs) are key schematics for industrial plants. In AutoCAD P&ID you can create these diagrams with a drag and drop interface of fluid and electrical schematic symbols. You can also build Instrument Lists and AutoCAD P&ID automatically updates your schematic drawings. Data-rich AutoCAD plant databases link your schematics with 3D models and plant item specs. Libraries and Blocks AutoCAD P&ID and Plant 3D come with many standard symbols and schematic components to streamline plant design. You also have the option to create custom symbols and save them to your own library folders. Block libraries help you effectively reuse components like pumps, vessels, piping specialties, instrumentation and structural items across your projects. CAM and Generating Toolpaths Computer-aided manufacturing (CAM) software converts 3D models into instructions for CNC machines like milling machines, lathes, routers, lasers and plasma cutters. The process of generating these toolpaths is called CAM programming. Some of the common toolpath types in CAM include: Contour toolpaths - Follow the contour of a part to machine the outside shape. Used for finishing and profiling operations. Pocket toolpaths - Clear material from inside a closed boundary to create a cavity or pocket in the part. Used for roughing internal areas. Drilling toolpaths - Add holes specified in the 3D model. Used for drilling holes. Thread milling toolpaths - Cut screw threads into a hole. Used for adding threads to holes. The basic CAM programming workflow involves: 1. Importing or creating 3D geometry in the CAM system. 2. Defining the stock boundaries. This represents the raw material block size. 3. Selecting tools from the tool library like end mills, ball end mills, drills. 4. Generating toolpaths - selecting geometry and choosing suitable toolpath types. 5. Simulating the toolpaths to detect collisions, inefficiencies and errors. 6. Post-processing the toolpaths to the language understood by the CNC machine. Simulations are critical to verify the toolpaths before machining the actual part. They help visualize the material removal process and identify issues like: Gouging - Tool extending below the target surface Undercuts - Areas missed by the tool Collisions - Tool intersecting with clamping fixtures or other obstacles Advanced CAM software can simulate material removal, tool wear over time and machine behavior to ensure efficient and error-free machining. Accurate CAM programming and simulation results in higher quality parts, safer machining and lower cycle times. Collaboration and Data Management Working on projects with large teams or managing large datasets requires collaboration tools to coordinate workflows. AutoCAD offers several features to facilitate collaboration and data management: Working with References and Xrefs External references (xrefs) allow you to link drawings rather than importing geometry. This enables collaborative workflows by allowing multiple designers to work on the same master drawing simultaneously. Xrefs also reduce file size and help organize complex projects. To work with xrefs you need to first create a drawing file that will be externally referenced. This xref can then be inserted into other drawings. You can control the visibility, binding, and other properties of the xref. Any changes made to the xref drawing file will automatically update in the host drawings. Collaboration Tools AutoCAD supports built-in collaboration tools and integrates with Autodesk cloud services: Autodesk Drive enables you to store and share both 2D and 3D designs from AutoCAD in the cloud. The Autodesk desktop connector synchronizes your AutoCAD designs and custom tool palettes with the cloud. Autodesk Anywhere helps coordinate design projects across extended teams. The Autodesk Viewer allows anyone to view 2D and 3D designs through a web browser. Vault Software Autodesk Vault provides advanced data management capabilities including version control, workflows, item lifecycles, and reporting. Vault helps organize, manage and track changes across a large collection of AutoCAD files. Key features include: File versioning - ability to track revisions and manage file history Access control - set user permissions Workflows - coordinate design review and approval processes Integration - connect with email, calendars, and other business systems Reporting - generate reports on project status, revisions, etc. Vault streamlines collaboration, especially for large teams and complex projects. It gives admins control over AutoCAD data while enabling designers to work efficiently. Rendering and Visualization Rendering and visualization in mechanical CAD are crucial for bringing designs to life and showcasing your work professionally. At this stage of your learning, you'll want to become familiar with materials, lighting, photorealistic rendering, and animation. Materials and Lighting Applying accurate materials like metals, plastics, glass, etc. and setting up proper lighting is the foundation of visualization. Learn how materials interact with different lighting types like point lights, spot lights, and ambient light. Understand how to control light intensity, color, falloff, and shadows. Advanced Rendering To take your renders to the next level, dive into photorealistic rendering techniques. Use raytracing for accurate reflections, refractions, shadows, and global illumination. Explore render engines like V-Ray for incredibly detailed and photorealistic results. Learn to adjust samples, noise thresholds, caustics, and indirect illumination for clean, professional renders. Animation Walkthroughs Bring your CAD designs to life with animation walkthroughs and fly-throughs. These are a powerful way to tell a visual story and showcase your designs. Learn camera animation techniques like panning, orbiting, dollying, and positioning camera paths. Understand editing keyframes on a timeline for smooth playback. Great animations take practice, so be patient and keep iterating. With advanced rendering and visualization skills, you can showcase your CAD work in the best possible light. Stunning images and animations will help you win clients and clearly communicate your designs. Automation and Customization One of the most powerful capabilities of AutoCAD is being able to automate repetitive tasks and customize the interface for enhanced workflows through scripting, macros, and LISP routines. This allows users to work more efficiently by eliminating redundant clicking and typing. Scripting and Macros Scripting in AutoCAD allows you to record commonly repeated commands and playback the script. This automates multi-step processes with a single click. Popular scripting options include: Action Macros - Record and save sequences of commands for playback later. Helpful for automating simple routine tasks. AutoLISP - Program custom functions using the LISP programming language. Widely used to automate CAD tasks and workflows. Visual Basic for Applications (VBA) - Similar to AutoLISP but uses Visual Basic syntax. Integrates with other Windows applications. ActiveX/COM - Allows interacting with AutoCAD objects using Visual Basic, C#, and other languages. Useful for advanced interoperability. Customizing Interface You can customize AutoCAD's interface, toolbars, menus and workspace to optimize it for your specific needs. Some popular ways of customizing AutoCAD include: Creating custom tool palettes with frequently used blocks, hatch patterns, etc. Customizing ribbon tabs and panels to quickly access the tools you use most. Setting up alternative workspaces optimized for common workflows. Adding custom menu macros to automate multi-step tasks. Customizing mouse buttons for quick access to commands. LISP Routines LISP (List Processing) allows users to write programs that automate complex CAD tasks. Some example uses: Performing repeating calculations. Extracting and processing drawing data. Modifying objects on the fly while working in your drawing. Creating custom dialog boxes and forms. LISP opens up an extensive range of customization possibilities to enhance productivity. With AutoCAD's robust automation and scripting options, you can significantly optimize and accelerate your design workflows. Investing time in learning these tools can provide huge time savings over the long run. Resources for Practice Becoming an expert at AutoCAD requires hands-on practice to reinforce your skills. Here are some recommendations for practice resources: Recommended Courses Udemy - CAD Exercises for 2D and 3D Practice LinkedIn Learning - AutoCAD Practice Drawings from Beginner to Advanced Skill share - AutoCAD Workbook with 50 Practice Drawings These courses provide step-by-step tutorial drawings and models that allow you to practice the AutoCAD skills covered in the lessons. They range from beginner to advanced level. Practice Drawings and Models CADalyst - Free AutoCAD Practice Drawings to Download Tutorial45 - 28 AutoCAD Exercises for 2D and 3D Practice CAD SetterOut - AutoCAD Practice Drawings Library These sites offer libraries of practice drawings and 3D models that you can download for free. They cover real-world examples like architectural plans, mechanical parts, civil diagrams, etc. Certification Prep Autodesk - Certified User and Certified Professional Practice Exams Udemy - AutoCAD Certified Professional Exam Prep Course Use these practice exams and courses to prepare for Autodesk's AutoCAD certification. They will help assess your skills and get you ready to pass the exams. Practice is essential for developing the muscle memory and speed needed to use AutoCAD efficiently. It also reinforces your knowledge by applying it to real design tasks. Take advantage of these resources to sharpen your skills on the way to becoming an AutoCAD expert.
- 30 Useful Keyboard Shortcuts for Speeding Up Your Mechanical CAD Workflow
Introduction Keyboard shortcuts are an indispensable tool that CAD professionals must master to maximize productivity and efficiency. While CAD software provides access to dozens or even hundreds of tools and commands, searching through menus and toolbars wastes precious time during the design process. Keyboard shortcuts allow rapid access to commonly used tools and streamline workflows by reducing hand movements between mouse and keyboard. Mastering shortcuts is especially crucial for disciplines like mechanical design and architectural drafting where speed and precision are paramount. This guide will provide an overview of the most useful keyboard shortcuts for mechanical CAD across several key areas: View Manipulation: Navigating the drawing canvas Drawing & Editing: Creating and modifying CAD geometry Dimensioning: Adding dimensions to drawings Annotation: Adding text and other annotations Organizing Drawings: Handling files and layouts Customizing Interface: Configuring the CAD environment Managing Files: Opening, saving, and exporting Command Line: Using the command line efficiently Advanced Features: Accessing specialized tools Having these shortcuts locked into muscle memory will enable CAD operators to keep their hands on the keyboard as much as possible. The boost in efficiency adds up to huge time savings over the course of a project. Even saving a few seconds per operation results in hours of gained productivity over time. That's why all CAD professionals should invest time up front to master these critical keyboard shortcuts. View Manipulation Shortcuts Being able to quickly manipulate your viewport is essential for efficient CAD work. Here are some of the most useful keyboard shortcuts for panning, zooming, rotating, and restoring views in CAD: Panning and Zooming Pan: Press and hold the center/middle mouse button while moving the mouse. This will pan your view without changing the zoom. Zoom In: Roll the mouse wheel forward. This zooms into your drawing around the cursor location. Zoom Out: Roll the mouse wheel backward. This zooms out of your drawing around the cursor location. Zoom Extents: Type `Z` then `E` and hit Enter. This fits your entire drawing in the viewport. Zoom Window: Type `Z` then `W` and select an area to zoom into. This lets you quickly zoom into a specific region. Rotating Viewport Plan View: Type `V` then `P` and hit Enter. This sets a top plan view. Isometric View: Type `V` then `I` and hit Enter. This sets an isometric 3D view. Restoring Previous Views Previous View: Type `VP` and hit Enter. This restores the previous viewport setting. Undo View Change: Type `VU` and hit Enter. This undoes the last view change. Using these handy view manipulation shortcuts will let you fluidly navigate your CAD drawing and boost your productivity. With some practice, you'll be able to pan, zoom, and rotate your viewport without even touching your mouse! Drawing & Editing Shortcuts The keyboard shortcuts for drawing and editing objects can save you a huge amount of time compared to clicking through the ribbon toolbar icons. Here are some of the most useful ones: L - Draw line C - Draw circle A - Draw arc P - Draw polyline REC - Draw rectangle PL - Draw polygon Once you've drawn an object, you can quickly select, move, copy or delete it: SPACEBAR - Repeats last command ESC - Cancel current command ENTER - Complete the command CTRL+C - Copy object CTRL+V - Paste object CTRL+X - Cut object DELETE - Delete selected object To move or copy selected objects: M - Move CO - Copy Some other useful editing shortcuts: E - Erase objects GR - Group objects UN - Ungroup objects B - Create block Using keyboard shortcuts to draw and edit is much faster than clicking through all the icons. With some practice, these will become muscle memory and seriously speed up your CAD workflow. Start with the basic shapes and build up from there. Dimensioning Shortcuts Adding accurate dimensions is critical for any CAD drawing. Mastering these keyboard shortcuts for dimensioning will speed up the process considerably. D - Toggles between dimensioning modes DI - Starts the dimension command and opens the dimension dialog box DIMCONTINUE - Continues the previous dimension command DIMSCALE - Changes dimensioning scale DIMREASSOCIATE - Associates or reassociates selected dimensions to objects or points on objects. Useful for fixing broken dimensions. DDEDIT - Edits dimension text and extension lines Customizing dimension styles is also key for maintaining consistency in your drawings. These shortcuts help modify dimension styles quickly: DDIM - Accesses the Dimension Style Manager to create, modify, override, and compare dimension styles. DIMGAP - Sets dimension line gap DIMTAD - Controls the vertical position of text in relation to the dimension line. DIMTXT - Specifies the dimension text height. With practice, these dimension and style shortcuts will become second nature, allowing you to add clear, accurate dimensions with just a few keystrokes. Mastering them is one of the fastest ways to boost productivity as a mechanical CAD designer. Annotation Shortcuts Annotations allow you to add text, leaders, and tables to your CAD drawings. Here are some useful keyboard shortcuts for annotating your designs: Text `MTEXT` - Opens the multiline text editor to add text annotations `DDEDIT` - Edits existing text `STYLE` - Opens the text style manager `MATCHPROP` - Matches text formatting Leaders `LEADER` - Adds leader lines to text `QLEADER` - Adds a multi-segment leader line `MLEADER` - Adds a multileader object with formatted text `MLEADEREDIT` - Edits existing multileaders Tables `TABLE` - Opens the Table Style dialog box `TABLEDIT` - Edits existing tables `BEDIT` - Enters block editor mode for editing attributes in a block table Using keyboard shortcuts for adding text, leaders, and tables can help speed up the annotation process. Practicing the shortcuts until they become muscle memory will boost productivity. Keep this reference list handy when drafting to utilize these time-saving annotation tools. Organizing Drawings In any robust CAD project, organizing your drawings is crucial for efficiency and collaboration. Here are some key shortcuts for creating layers, managing layouts, printing, and more. Layers Layers allow you to organize different objects in a drawing. For example, you may have separate layers for walls, electrical, plumbing, etc. Some useful layer shortcuts include: `LA`: Layer properties manager `LT`: New layer You can quickly switch between layers with `Ctrl+PgUp` and `Ctrl+PgDn`. Layouts Layouts let you create separate views of your drawing for printing or exporting. Helpful shortcuts include: `MS`: Opens sheet set manager `PS`: Page setup manager `Ctrl+Tab`: Cycles through layout tabs Printing When it's time to print, these shortcuts come in handy: `Ctrl+P`: Opens the Print dialog box `Ctrl+Shift+P`: Plots the current viewport/layout `Ctrl+Alt+P`: Options dialog box for plot Collaborating To support collaboration and file management: `DWGPREFIX`: Sets drawing name prefix `FilesTab`: Opens file explorer `eTransmit`: Packages drawing files for easy sharing By mastering these organizational shortcuts, you can manage complex CAD projects more efficiently. Customizing Interface A key to boosting productivity in AutoCAD is customizing your interface and workspace to match your workflow. There are several options for customizing your AutoCAD environment: Organizing Toolbars and Ribbons AutoCAD's ribbons can be customized to show only the tools you use most often. Right click on any ribbon tab and select 'Customize Ribbons' to add and remove tools from the ribbon. You can also create your own custom ribbon tabs to group specific tools. To customize toolbars, right click on any toolbar and select 'Customize'. Here you can add, remove, and rearrange toolbar buttons. Consider removing redundant tools and placing your most used commands front and center. Tailoring Workspaces Workspaces allow you to save customized ribbon and toolbar layouts for quick access. To save a workspace, go to the Workspace Switching button in the status bar and select 'Save Current As'. Give your workspace a name and it will now be accessible anytime from the workspace menu. Create separate workspaces optimized for different tasks like drafting, 3D modeling, rendering, etc. Switch between them instantly for a workflow boost. Customizing Shortcuts You can customize keyboard shortcuts for any command within AutoCAD. Go to Tools > Customize > Keyboard tab to view and modify key assignments. To change a shortcut, select the command, enter a new shortcut key, and click 'Apply'. Leverage shortcuts to access frequent commands rapidly. Consider shortcuts for view controls, draw/modify tools, annotation commands, etc. Start with essentials, then build up your shortcut repertoire over time. With some personalization, you can transform AutoCAD's interface into a highly efficient environment tailored for your unique needs. Invest time in customization for a long-term productivity increase. Managing Files Managing files efficiently is critical for an optimized CAD workflow. Here are some keyboard shortcuts for opening, saving, converting, and referencing external files in your CAD software: Opening and Saving Drawings Ctrl + O - Open an existing drawing Ctrl + S - Save the active drawing Ctrl + Shift + S - Save drawing under a new name or location By memorizing the open and save shortcuts, you can access your files much faster than navigating through menus and dialog boxes. Converting File Formats Ctrl + I - Import a file and convert it to a .dwg Ctrl + E - Export the active drawing in a different file format like .pdf or .dxf Converting between file formats is common in CAD work sharing. These keyboard shortcuts eliminate the need to open converter utilities separately. Attaching External References Ctrl + Shift + D - Attach an xref to the drawing Ctrl + Shift + A - Detach or unload an xref Ctrl + Shift + R - Reload or refresh an xref Xrefs allow you to link data between files. The xref shortcuts help you access and update external references without disrupting your workflow. By learning keyboard shortcuts for managing files, you can significantly reduce the time spent navigating dialog boxes. These shortcuts allow speedy access to your CAD drawings and associated files. Command Line Shortcuts The command line is a powerful tool in CAD that allows you to quickly invoke commands by typing their name or alias. Mastering the command line can significantly boost your productivity. Here are some tips for using the command line effectively: Learn command aliases - Most commands have a shortened alias you can type instead of the full name. For example, "PL" for "POLYLINE", "CP" for "COPY". Using aliases can save a lot of keystrokes. Right-click tricks - Right-clicking in the command line will display a list of recently used commands. You can quickly re-run a command this way. Press F2 - This will toggle the command line display on and off. Keep it open to use commands faster. Type first letters - When a command window pops up, you usually only need to type the first 1-3 letters of the command name for it to be recognized. Tab completion - If you only type the first few letters of a command name and press TAB, the command line will auto-complete the rest for you if there is a unique match. SPACEBAR - Hitting spacebar will repeat the last command entered. This can save you tons of re-typing. UP arrow - Press the UP arrow key to cycle back through the history of commands recently used. Learn command syntax - Many commands have options and flags you can add as arguments in the command line. Learn syntax like "/ISO" "/P" "/A" to control commands. Mastering these command line techniques will make you a power user. Keep the command line open and your fingers on the keyboard to boost productivity. Advanced Features For expert CAD users looking to take their skills to the next level, AutoCAD offers advanced capabilities through scripting, customization, and plugins. Scripting AutoCAD supports several programming and scripting languages that allow you to automate repetitive tasks. These include: AutoLISP - This is AutoCAD's native scripting language. AutoLISP scripts can automate tasks, modify the interface, and create custom commands. Visual LISP - A version of AutoLISP with an editor that provides syntax highlighting and debugging tools. Makes it easier to write, modify and troubleshoot AutoLISP scripts. VBScript - A lightweight programming language from Microsoft. VBScript can be used to create scripts for batch processes and automate workflows. .NET - For advanced scripting capabilities, the .NET framework allows creating add-ons and apps using C# or VB.NET. To get started with scripting, check out AutoCAD's documentation on programming interfaces. Start small by recording actions with the Action Recorder tool and reviewing the resulting scripts. Customization Expert users can customize the AutoCAD interface and features by: Creating custom ribbons, toolbars and menus Assigning shortcuts and aliases to automate workflows Setting up action macros to record repetitive tasks Developing custom line types, hatches, templates and more Modifying system variables to tweak settings Installing third-party plugins Dig into the Customize User Interface (CUI) to customize tool locations, modify ribbons and menus, and import/export customization files. Plugins and Add-Ons An active developer ecosystem offers plugins that add new functionality to AutoCAD: BIM add-ons - Expand building information modeling capabilities. Rendering tools - Enhance visualization with physically-based rendering. Parametric design - Introduce constraints and relationships between objects. 3D printing plugins - Additional tools for 3D printing workflows. Popular plugin communities include Autodesk App Store, CAD Studio, The Swamp, and the Object ARX SDK. Learning to tap into AutoCAD's customization and extensibility will reveal advanced capabilities beyond typical out-of-the-box use.
- 5 Emerging Trends Shaping the Future of Mechanical CAD Industry
Introduction The mechanical CAD (computer-aided design) industry is a critical driver of innovation across countless sectors and products. Mechanical CAD software provides the essential tools for engineers and designers to conceptualize, model, analyze and document mechanical components and products. From consumer electronics, to medical devices, to industrial equipment, almost every engineered product relies on mechanical CAD systems during development. As a mission-critical tool for engineers, it is vital that mechanical CAD software keeps pace with emerging technologies and evolves to enable new design possibilities. The mechanical CAD industry is currently undergoing significant changes, driven by major technological advancements in areas like artificial intelligence (AI), additive manufacturing, cloud computing and more. There are 5 key trends poised to transform mechanical CAD software, workflows and deliver new capabilities that will shape the next generation of mechanical design and manufacturing: Automation & Smart CAD Systems The automation revolution is coming to mechanical CAD, ushering in a new era of intelligent and self-optimizing systems. Advances in robotics, machine learning and generative design are enabling CAD software to take on more complex and repetitive design tasks with minimal human input. One key area of innovation is the integration of AI and automation into the CAD workflow. Rather than step-by-step human direction, new AI-powered CAD systems can analyze design goals and manufacturing constraints to explore millions of design iterations. Machine learning algorithms discern optimal configurations, geometries, and material selections to meet performance requirements with the least amount of physical testing and prototyping. Generative design takes this a step further by enabling designers to input parameters like function, materials, manufacturing methods, and cost - then leverages computational power to output the most efficient design. This automated trial-and-error process unlocks novel solutions that human designers may never have conceived of. Intelligent CAD systems with self-optimizing capabilities can even monitor real-world performance data through IoT sensors and continuously adjust designs for improved efficiency and sustainability. As these smart CAD systems mature, they will minimize the repetitive grunt work of drafting and free up engineers to focus on innovation. The integration of automation, AI and generative design promises to reshape mechanical CAD into a highly intelligent tool capable of accelerating development cycles and unlocking unprecedented efficiencies. While human oversight remains critical, reducing repetitive tasks allows engineers to focus their expertise on imagination, strategy and pushing boundaries. 3D Printing & Additive Manufacturing The rise of 3D printing and additive manufacturing is one of the most disruptive emerging trends influencing mechanical CAD. 3D printing enables the production of highly complex geometries and customized parts that would be impossible or impractical with traditional manufacturing methods. Parts can be printed on demand in small batches without the need for expensive tooling or molds. This enables distributed manufacturing and has significant implications for supply chains and inventory management. With 3D printing, mechanical engineers are no longer constrained by the limitations of subtractive manufacturing. They can design organic shapes, lattices, and complex internal structures to optimize the strength, weight, and functionality of components. 3D printing unlocks new design possibilities and enables the production of parts with enhanced performance characteristics. Parts can be printed with gradients and multiple materials to meet precise mechanical requirements. The environmental sustainability of 3D printing is also notable. Additive manufacturing generates far less material waste than traditional manufacturing which relies on cutting away excess material. 3D printing uses only the required amount of material for each part. Unused powder can be reused, further reducing material waste. Distributed 3D printing reduces emissions from shipping and transportation. As the technology advances, 3D printing with recycled plastics and sustainable bio-materials will provide further sustainability benefits. In summary, 3D printing and additive manufacturing will continue transforming mechanical design by enabling complex geometries, distributed production, and more sustainable manufacturing. It expands the design possibilities for mechanical engineers and allows them to optimize parts for advanced performance in ways not feasible with traditional methods. Adoption of 3D printing will only accelerate as the technology matures. Cloud-Based CAD: The Benefits of Shifting Mechanical Design to the Cloud The cloud has transformed many areas of work, and mechanical CAD is no exception. Cloud-based CAD enables designers to access CAD software and 3D models anywhere through a web browser. This means mechanical engineers can collaborate and work on designs from any location, rather than being tied to specific workstations with CAD software installed. Some key benefits of utilizing cloud-based CAD include: Access anywhere - With cloud-based CAD, designers can log in from any computer or mobile device with an internet connection to work on designs. Files and projects are available anywhere without needing to transfer data or manage multiple versions. Real-time collaboration - Cloud platforms make it easy for distributed teams to collaborate on designs in real-time. Engineers across multiple locations can simultaneously view and edit the same CAD model and exchange feedback. Data backup and security - Cloud CAD providers maintain data backups, disaster recovery systems and stringent security, alleviating concerns over losing work or designs getting compromised. Cost savings - Cloud CAD systems are typically subscription-based, eliminating the high upfront costs of buying and maintaining CAD software and hardware. These savings can be reinvested into design work. Simplified version control - Tracking changes and managing file versions is seamless with cloud-based CAD. Automatic version histories reduce headaches when iterating on designs. As high-speed internet access spreads globally, expect more design firms to leverage the flexibility of cloud-based CAD. This shift enables teams to collaborate efficiently across distances and reinvents traditional CAD workflows. With on-demand access to computing resources, cloud platforms remove technology limitations for designers and engineers. Digital Twins and CAD Optimization Digital twins are one of the most transformative technologies impacting mechanical CAD design and optimization. A digital twin is a virtual representation of a physical product or system that uses real-time data and simulations to mirror the life of its physical counterpart. Digital twins are poised to revolutionize how mechanical engineers design, optimize, and maintain products. In the CAD process, digital twins allow for the creation of a linked simulation model that responds to changes in the CAD design in real-time. As mechanical engineers modify their CAD models, they can instantly see the impact on the digital twin simulation. This creates a feedback loop that allows rapid design optimization. Additionally, when coupled with real-time sensor data from IoT connected products in the field, digital twins become even more powerful. The digital twin model can ingest live data coming from thousands of sensors on installed products. This data can enhance the accuracy of the simulations to truly mimic real-world performance. Engineers can then tap into the digital twin to optimize in-service products. For example, an automotive engineer could link the digital twin of a vehicle design to IoT data from all the deployed cars. This would give them unprecedented insights into how the vehicles are actually being used in the real world. The engineer could use this to modify the digital twin and CAD design to improve issues that are occurring in the field. This enables a major shift left in the design process - optimization after product launch. Digital twins stand to help companies substantially accelerate mechanical CAD workflows, improve product performance, and enable predictive maintenance for reduced downtime. Adoption of digital twins and linkage to live IoT data will be a key trend propelling the CAD industry forward over the next 5 years. Artificial Intelligence & Machine Learning Fuel Innovation Artificial intelligence (AI) and machine learning are having a major impact on mechanical CAD, enabling generative design, process optimization, and predictive insights. Rather than simply assisting designers, AI is beginning to take a more active role in the creative process. Generative design powered by AI allows designers to specify parameters and goals for a part, while algorithms generate thousands of design options optimized for factors like weight, strength, manufacturability, and cost. This accelerates the design process and unlocks solutions that designers may not have conceived of manually. AI techniques like deep learning and neural networks can analyze usage data on existing products to gain a holistic understanding of real-world performance. These insights allow CAD systems to optimize future designs by predicting how changes will impact key metrics. For example, airflow and temperature data could inform generative algorithms to create designs optimized for cooling capacity. By automating repetitive, low-level tasks, AI frees up designers to focus their efforts on more creative, strategic work. Machine learning algorithms continually improve by learning from each iteration, allowing CAD systems to provide an increasingly intelligent and responsive user experience. Rather than replacing human designers, AI enhances designers' abilities and allows them to operate at a higher level. As AI capabilities grow more advanced, they will work synergistically with designers to rapidly develop innovative products optimized for cost, utility, and sustainability. Sustainability and Green Engineering Sustainability is becoming an increasingly important consideration in the mechanical CAD industry. CAD tools now allow engineers to evaluate and optimize the environmental impact of their designs. Simulations can be run to assess factors like energy usage, emissions, and material waste. This empowers designers to make more informed decisions when developing sustainable products. Many CAD platforms now incorporate design for sustainability principles directly into the software. This includes the ability to select sustainable materials, reduce part counts, and minimize waste. Designers can leverage lightweighting techniques to cut down on material usage. Tools also exist to optimize packaging and logistics. Generative design techniques enabled by AI allow designers to develop novel, organic shapes that are material efficient. By using algorithms to iterate through designs, CAD software can automatically identify geometries that minimize material volume while preserving strength. The ability to assess full lifecycle impacts is also advancing. CAD integrates with PLM software to connect design data to sustainability metrics like end-of-life recycling rates. By taking a holistic view across the entire product lifecycle, engineers can develop optimal concepts from the outset. Mechanical CAD platforms continue to expand capabilities related to sustainability. Usage of sustainable design practices early in the engineering process is key for developing eco-friendly, energy efficient products. CAD tools empower designers to understand tradeoffs and make environmentally conscious decisions. Adoption of green engineering principles will be crucial as businesses and consumers demand products with improved sustainability. Collaborative Design Workflows The era of the solo mechanical CAD designer working in isolation is fading fast. Modern CAD tools now enable real-time collaboration on designs across globally distributed teams. Rather than emailing CAD files back and forth and struggling to sync up, engineers can now co-design products in real-time regardless of where they are located. Cloud-based CAD platforms allow for simultaneous collaboration on designs from multiple locations. This facilitates rapid iteration as multiple team members can provide input on the fly. Design changes and comments from collaborators are instantly reflected in the CAD environment. Seamless integration with messaging and video chat features enables fluid team discussions directly within the CAD interface. Role-based access controls also allow organizations to manage user permissions when collaborating on sensitive CAD designs. Controls can restrict access to certain features, parts or products depending on user roles and security clearance. This ensures confidential aspects of product designs are kept secure while still enabling collaboration on a need-to-know basis. The rise of collaborative CAD promises to break down silos and accelerate innovation cycles. With real-time co-creation of designs and role-based access management, mechanical engineers can finally collaborate seamlessly on products in the digital age. Rapid Development & Production The demand for faster product development cycles and rapid manufacturing capabilities is driving innovations in mechanical CAD tools and workflows. Generative design and topology optimization are two technologies that are enabling much faster initial concept designs compared to traditional CAD methods. Generative design uses artificial intelligence and optimization algorithms to quickly generate design alternatives that meet desired parameters and constraints. By simply defining goals like size, weight, strength, manufacturability, performance, and cost, generative design software can produce numerous viable options to choose from. This allows mechanical engineers to bypass much of the repetitive, iterative conceptual work and jump right to refining a developed design. Topology optimization is another innovative technique for structural design optimization that produces organic, efficient shapes tailored to load conditions and constraints. By optimizing the distribution of material, topology optimization minimizes weight while ensuring strength requirements are met. This can lead to complex geometries that are impossible to conceptualize manually. These new capabilities are transforming the early stages of the design process. What once took days or weeks of modeling can now be accomplished in hours or minutes. This enables faster iteration, testing, and selection of the optimal design. The whole product development timeline is further accelerated by improved interoperability between CAD, CAM, CAE, and other engineering software tools. Modern CAD platforms have seamless integration and data transfer capabilities with downstream manufacturing applications. This facilitates moving from virtual prototyping to physical production faster than ever before. With real-time synchronization of CAD geometry and CAM toolpaths, manufacturers can initiate fabrication quickly without waiting for data translation and revisions. By linking CAD models with CAE stress analysis results, designs can be rapidly optimized and refined before manufacturing. Connecting CAD with PLM and ERP systems enables an integrated product lifecycle management workflow from conception to delivery. Together, these innovations in generative design, seamless tool integration, and rapid manufacturing are compressing development timelines and allowing mechanical engineers to deliver high quality products faster and more efficiently than traditional methods. This improved agility provides major competitive advantages to manufacturers who leverage these leading-edge technologies. Conclusion The mechanical CAD industry is entering an exciting new era shaped by emerging technologies that will transform how products are designed, optimized, manufactured, and used. As we have explored, trends like AI, 3D printing, IoT, and cloud computing are poised to disrupt the status quo and create new opportunities for innovation in mechanical engineering. Companies that want to gain a competitive edge need to seriously consider how they can leverage these innovations. Implementing cutting-edge CAD systems with automation, generative design, and real-time collaboration features will allow businesses to significantly accelerate their product development. Combining the powers of CAD, PLM, IoT, and digital twins will enable previously impossible levels of optimization through the entire product lifecycle. And localized manufacturing via 3D printing will permit faster production with less waste and environmental impact. While adopting new technologies always comes with challenges, the potential benefits make the effort worthwhile. Companies that embrace these trends early will be best positioned to revolutionize mechanical design and transform themselves into organizations ready for the future. The outlook for the mechanical CAD industry is bright, as long as users are willing to embrace the change brought by these groundbreaking innovations. Businesses that leverage them now will gain a decisive competitive advantage in their industries.
- 8 Key Principles for Designing Machined Parts Using CAD Software
Introduction Computer Aided Design (CAD) software allows engineers and designers to create detailed 3D models and technical drawings of machine parts and other components. The use of CAD has become an indispensable tool in manufacturing and engineering workflows. CAD models designed for fabrication using computer numerical control (CNC) machining must follow specific principles and best practices. Well-designed CAD models make the CNC programming and machining process quicker, more efficient, and higher quality. This guide covers the 8 key principles that engineers should follow when using CAD software to design parts intended for CNC machining. By understanding these fundamentals, you can optimize your CAD models to avoid common issues, minimize machining time, reduce cost, and achieve dimensional accuracy. Whether you are designing precision turned parts, complex castings, plastic injection molds, or machined components, adhering to these CAD best practices will lead to efficient, optimized designs ready for flawless CNC fabrication. Understand CNC Machining CNC stands for Computer Numerical Control and refers to machining processes that are controlled using computers and CAD/CAM software. Whereas conventional machining relies on manual operation of machine tools, CNC machining uses programmed computer controls to automate fabrication. The basic CNC machining process works as follows: 1. The part design is created using CAD (Computer-Aided Design) software. Common CAD programs used for CNC machining include SolidWorks, AutoCAD, and Inventor. 2. The CAD model is converted into CNC code through CAM (Computer-Aided Manufacturing) software. The CAM program analyzes the CAD model and generates code telling the CNC machine how to move. 3. The CNC code is then loaded onto the CNC machine controller. CNC machines read g-code and m-code instructions to guide the machine tool motion. 4. The machinist loads the raw material into the CNC machine and initiates the program. Common CNC tools include mills, lathes, routers, grinders, drills, lasers, plasma cutters, and waterjets. 5. The CNC machine uses rotating cutting tools or other processes to cut away material based on the toolpaths defined in the CNC program. 6. The finished part is unloaded once the automated machining process is complete. Some secondary finishing may be required. In summary, CNC machining automates fabrication by using computer numerical control programs to direct machine tools. This improves precision, repeatability and efficiency compared to manual machining. Understanding the CAD to CNC process is key for designing optimized parts. Improve Engineering Drawings Creating clear, effective engineering drawings is critical for designing machined parts in CAD. Here are some key principles for improving your drawings: Include dimensions only for critical and measurable features - Eliminate any superfluous dimensions that are not needed to fully specify the part. Focus on adding just enough dimensions to describe the necessary size, position and geometric relationships between features. Add hole tapping needs including thread size and depth - Specify any tapped holes by calling out the thread size and required engagement depth. This ensures the machinist will create holes ready for tapping at the required sizes. Consolidate call-outs when there are multiples of the same feature - When a view shows multiple copies of the same feature, group them in a single note instead of multiple repetitive notes. For example, note "All 4 holes tapped 1/4-20 x 1/2 deep" instead of 4 separate call-outs. Orient views to minimize view changes - Orient plan views consistently to avoid flipping the part. This makes it easier to visualize the true orientations. Add centerlines to locate holes and features - For holes or features that are not tied to other dimensions, use centerlines to locate their positions accurately. Following these drawing best practices will ensure your CAD engineering drawings contain just the right information needed for manufacturing, minimizing complexity, errors and confusion. Well-executed drawings efficiently communicate design intent to streamline production. Communicate Assembly Intent Clear communication of assembly intent in the CAD model is crucial for efficient machining. The drawing should provide all the information needed for fabrication without ambiguity. Include Critical Part Numbers Part numbers are essential for identifying each component correctly during manufacturing. Number all parts sequentially or according to internal part numbering conventions. Add the part number prominently next to each part in the drawing. Avoid cluttering the drawing with part labels, but make sure each part can be clearly identified. Exclude Non-Critical Operations Secondary operations like tapping holes or adding threads often do not need to be specified in the initial drawing. Providing unnecessary call-outs adds clutter and potentially confusing information. Only include critical specifications needed for the initial fabrication. Streamline Explanation of Intent The drawing should be unambiguous about assembly order and relationships between components. Arrows, exploded views, numbered sequences or keys can clarify component relationships and assembly order. Remove any redundant or excessive dimensioning that does not aid assembly intent. Simplify and streamline the information for clarity. By focusing on critical part numbers, minimizing unneeded secondary operation notes, and clearly communicating assembly intent, the CAD drawing will enable efficient fabrication of the designed parts. Remove ambiguity by providing only essential specifications required for machining each component accurately. Avoid Over-Dimensioning Over-dimensioning your CAD designs can lead to several issues during machining. Every dimension added to the drawing requires extra time for both the CAD work and CNC programming. Excess dimensions also clutter the drawing, making it more difficult to discern the truly critical features. Parts should only be dimensioned to the accuracy level needed for correct function. For example, a locating hole for a screw may only require +/- 0.5 mm accuracy, while a bearing shaft may need +/- 0.05 mm. Consult machining handbooks and standards guides for recommended tolerances based on the material, feature type, position, and function. Adding too many tolerances is a form of over-dimensioning. Tight tolerances increase costs and reduce material removal rates in CNC machining. Only critical features that impact form, fit and function require specific callouts. Non-critical aesthetic features can use default tolerances. Consider both the end use requirements and capabilities of your machining process when assigning tolerances. Following industry standards helps avoid over-dimensioning. Standards provide guidance on which features typically need dimensioning versus applying default values. They also give you recommendations for standard tolerance grades to call out. Using standard practices improves manufacturing clarity and efficiency. Overall, focus dimensions and tolerances only on critical features and specifications. Remove any redundant dimensions that over-constrain the design. Lean drafting focuses on conveying the essential information clearly and concisely. This allows smoother transitions from CAD to CNC machining. 6. Design for Machinability Machined parts must be designed in a way that allows CNC tools to physically cut and create the required shapes. Following some key rules of machinability can optimize designs for efficient and successful CNC fabrication: Avoid Excessively Thin Walls - Thin walls that are less than 1/4 inch thick can easily warp and bend during machining. They also provide inadequate surface area for tools to cut properly. For most materials, wall thicknesses should be designed to at least 1/4 inch. Eliminate Undercuts - Undercuts create overhangs and areas that cannot be reached by straight CNC cutting tools without special fixtures. Avoid undercuts in the design. Avoid Tight Internal Radii - All internal edges should have radii added to them. Tight sharp internal radii are difficult to cut and can fracture. Use radii of at least 1/8 inch. Avoid Intersecting Holes - Wherever possible, design holes to have some minimum distance between them. Intersecting holes are challenging to machine. Design with Simple Geometric Shapes - Complex free-form shapes, knots, and spirals are unmachinable with basic CNC tools. Design using combinations of simple machinable shapes like rectangles, circles, cylinders, etc. Following these rules will ensure designs are optimized for efficient, low-cost, and problem-free CNC machining. Eliminating thin walls, undercuts, tight radii, intersecting holes, and complex shapes goes a long way in machinability. Control Tolerances Overly tight tolerances can significantly increase manufacturing time and cost. This occurs because achieving high precision requires slower feed rates, decreased material removal, and additional finishing work. When designing parts in CAD, do not specify tolerances tighter than the standard guidelines for your material and cutting method. Common tolerance grades are: Aluminum parts cut on a CNC mill: ±0.005 in to ±0.02 in Steel parts cut on a CNC mill: ±0.002 in to ±0.01 in Aluminum parts cut on a CNC lathe: ±0.001 in to ±0.005 in Steel parts cut on a CNC lathe: ±0.0005 in to ±0.002 in In general, aim for the largest workable tolerance. Only use tighter tolerances for critical features like locating pins, bearing surfaces, and mating components. Relax tolerances on non-critical aesthetic surfaces whenever possible. Review your industry's accepted tolerance standards and always design parts with standard tolerance grades. Over-specifying tolerances causes unnecessary machining costs and reduces shop productivity. Minimize Unnecessary Features When designing parts in CAD for CNC machining, it's important to minimize any features that are not functionally required in the final design. While aesthetic features like rounds, chamfers, or complex curves may look nice, they require additional programming and machining time that drives up costs. The key is to strike the right balance between meeting functional needs and minimizing machining time. Only add aesthetic features if they serve a functional purpose, like improving part strength, facilitating assembly, or meeting end-use requirements. But avoid adding extra curves, holes, patterns, or textures just for appearance when they provide no functional benefit. For example, if a part will be hidden inside an assembly, a plain blocky design will machine faster than one with rounds and scallops on every surface. Or if a part requires holes to mount it, aim for the simplest hole pattern that enables assembly rather than an intricate array of unnecessary holes. In CAD software, it can be tempting to explore complex designs. But each curve, contour, hole, and surface requires additional toolpaths and machining time on a CNC machine. So developing a basic functional design first, then only adding secondary features as needed for function or appearance, helps to minimize costs. A clean, elegant design aligned to manufacturing and functional needs takes skill but will machine efficiently. Optimize Cavity Depth-to-Width Ratios When designing machined parts in CAD, pay close attention to the depth-to-width ratio of any cavities, pockets, or holes. An optimal ratio allows for efficient machining by ensuring the cutting tools can fully access the entire feature. As a general rule, aim for depth-to-width ratios between 1:1 and 3:1. A 1:1 ratio means the depth equals the width. A 3:1 ratio means the depth is three times the width. These ratios provide enough clearance for cutting tools to machine the entire cavity. For example, if a pocket is 2 inches wide, its depth should be between 2-6 inches. If the depth exceeds 6 inches, it becomes difficult for cutting tools to reach the bottom of the pocket. The tools can deflect, reducing accuracy and leaving behind unwanted material at the bottom. For holes and slots, a depth around 2-3 times the diameter is ideal. This prevents the tools from rubbing or binding along the sides as they drill or mill into the feature. In some cases, a ratio greater than 3:1 may be necessary based on part functionality. But this increases machining time, cost, and risk of tool failure. When possible, redesign the part to allow an optimal ratio. For deep cavities, consider creating access holes, scallops or slots. This provides an entry point for tools to start machining the bottom surface. Then secondary operations can finish the full cavity design. By optimizing depth-to-width ratios in the CAD design phase, you can dramatically improve the machining process. Well-designed ratios minimize tool strains, prevent tool breakage, reduce cycle times, improve accuracy and lower costs. Conclusion Designing parts for CNC machining using CAD software requires attention to detail in the modeling and drawing process. By following the key principles outlined in this guide, you can create optimized designs ready for efficient and accurate machining. To summarize, focus first on the fundamentals of engineering drawings like dimensions, tolerances, and annotations. Communicate the complete assembly intent through part numbers, callouts, and assembly drawings. Avoid common oversights like over-dimensioning, under-dimensioning, or unrealistic tolerances. Understand the basics of CNC machining and how your CAD model will be translated into machined parts. Design your parts specifically for manufacturability, considering limitations of CNC tools and materials. Control tolerances to balance costs while still meeting design intent. And optimize features like wall thicknesses and cavity ratios for accessibility and machining time. With practice and application of these guidelines, you'll be able to design CAD models that machine like a dream. Your parts will go from concept to physical reality quickly, accurately, and efficiently. By mastering CAD for CNC, you gain creative freedom in design along with confidence that your parts will machined right the first time.
- 25 Practical Exercises to Sharpen Your Mechanical CAD Proficiency
Introduction CAD (computer-aided design) software allows engineers and designers to create 2D technical drawings and 3D models for architectural, engineering, and manufacturing projects. Developing strong CAD skills takes practice and repeated use of the tools and features within the software. These exercises are designed to help you master common CAD workflows, refine your technique, and become an expert CAD user. This guide provides 25 practical real-world CAD exercises to build up your proficiency. The exercises cover core skills like 2D drafting, 3D part modeling, rendering, and documentation. More advanced exercises incorporate reverse engineering, collaboration tools, simulation, and emerging technologies. The exercises are organized by skill level and category: Beginner: Exercises focused on 2D drafting fundamentals, 3D modeling basics, and documentation. Designed for new CAD users. Intermediate: Exercises to build more complex 3D parts and assemblies, architectural visualization, and product prototypes. Recommended for users with some CAD experience. Advanced: Exercises involving reverse engineering, mesh editing, collaboration, and presentations. For experienced users ready to expand their skillset. Each exercise includes step-by-step instructions, tutorial files, examples, and tips to complete the project successfully. Mastering these real-world exercises will give you the confidence and skills to take on professional CAD projects and accelerate your workflows. Let's get started! 2D Floor Plans Drafting floor plans is one of the most common uses of 2D CAD. Being able to quickly layout and visualize a space in 2D is an essential skill. Start by sketching a floor plan of your own home or apartment. This will allow you to practice measuring rooms, hallways,doors and other features. Pay attention to the overall dimensions and proportions as you draft the floorplan. Add details like windows, doors, stairs and fixtures once you have the basic layout established. After completing a floor plan of your residence, try rearranging the furniture layout. Experiment with different configurations and walkthrough the plans to see if they work. Consider sightlines, traffic flow, and conversation areas as you refine the layout. Another key exercise is drafting electrical and lighting plans. This involves mapping out receptacles, switches, lighting fixtures and any other powered devices. Use standard electrical symbols and callouts as if it were for an electrician. Consider code requirements for placement and spacing. For more practice, draft floor plans for a variety of building types - offices, stores, restaurants, classrooms etc. Sketching first on grid paper can help visualize proportions. Take measurements from example floor plans or make reasonable assumptions. This will build experience quickly. As your drafting skills improve, focus on speed and efficiency. Time yourself to complete plans accurately under tight deadlines. Learn keyboard shortcuts to maximize productivity. Develop clean, standardized styles for elements like doors, textures, fixtures etc. With regular practice, 2D floorplans will become second nature. Mechanical Part Modeling Modeling mechanical parts like gears, fasteners, brackets, and basic machine components will build your proficiency in CAD fundamentals. Mastering these fundamental shapes will enable you to design more complex parts and assemblies down the line. Model Basic Gears Start by modeling simple spur gears with different numbers of teeth. Pay attention to the pitch diameter, outside diameter, tooth profile, and hole. Once you feel comfortable, try your hand at bevel, worm, and other gear varieties. Referencing schematics or photos is a great way to model real-world gears accurately. Create Threaded Fasteners Practice modeling threaded hardware like bolts, nuts, screws, and threaded rods. Pay special attention to capturing the complex thread geometry accurately. Start with simple external thread forms, then work your way up to more intricate internal threads and fastener head shapes. Design Mounting Brackets Mounting brackets come in endless configurations to position, align, and affix components. Modeling brackets will teach you sketch constraints, extrusions, cuts, and fillets. Aim for appropriate dimensions and clearances based on standard hardware sizes. Think about Dienability and how parts must fit together. Build Basic Machine Components Construct simple examples of bearings, shafts, pins, keys, springs, and other common machine elements. Refer to manufacturer catalogs for dimensions and configurations. Pay attention to the mating relationships between components. Modeling to precise tolerances will ensure your parts align properly in assemblies. With practice modeling these fundamental part types, you'll establish a strong foundation in mechanical CAD skills. Don't be afraid to explore and experiment on your own too. Referencing existing designs is a great way to ensure real-world accuracy. 3D Architectural Visualization Architectural visualization is one of the most valuable uses of 3D CAD software. Being able to create photorealistic renderings of building exteriors and interiors is crucial for design presentations to clients. Start by modeling simple geometric shapes like walls, windows, doors, stairs, and furniture. Pay attention to accuracy and scale. Once you have the basic shapes built, focus on adding architectural details. Model railings, trim, moldings, tile patterns, wall textures, and everything needed to make the building feel real. Think about lighting and add windows, lamps, sconces and other light fixtures. To take it to the next level, create night and day versions of the exterior. Build the surrounding landscape with plants, trees, fences, roads, cars, and people. Show interior and exterior colors, furnishings, and decor. With practice, you can render strikingly realistic architectural visualizations and floor plans. Use artistic flair to showcase your building designs. This is one of the most impressive capabilities of 3D CAD. Some architectural modeling exercises to try: Model your house or apartment interior and exterior Design your dream home with a pool, landscape, and furnishings Create a commercial building like a store, restaurant, or office Build a historical structure like a castle, mansion, or monument Construct a fantasy building, like Hogwarts from Harry Potter Make a futuristic structure with dramatic architecture The architectural possibilities are endless with CAD software. Constructing buildings from the ground up will give you valuable experience and impressive 3D models for your portfolio. Product Prototyping Bringing a new product idea to life is an exciting application of CAD skills. Start by conceptualizing and sketching out your design. Consider details like size, shape, materials, functionality and aesthetics. Once you have an initial concept, start modeling it in CAD. Construct the individual parts and assemblies needed to create your product prototype. Refine the design through multiple iterations, making tweaks and adjustments to optimize the form and function. Test your CAD prototype digitally by simulating real world conditions. Check for potential design flaws using finite element analysis tools. Assess manufacturability by experimenting with different fabrication techniques. Build physical prototypes to evaluate ergonomics, durability, and other tangible characteristics. Use methods like 3D printing, CNC machining or injection molding to produce sample parts. Gather feedback and reiterate on the design until you arrive at an optimal finished product. Creating a product from idea to prototype is a multifaceted challenge. But the CAD skills you gain from this exercise will prove invaluable for bringing innovative new products to life. Reverse Engineering Reverse engineering is the process of taking an existing object or product and analyzing it to recreate it via CAD. Here are some of the best ways to practice reverse engineering exercises: Import a 3D Scan With the rise of photogrammetry and affordable 3D scanning devices, importing a 3D scan into CAD software has become a very common reverse engineering technique. To practice: Scan an object using a 3D scanner or photogrammetry software like Autodesk ReCap. Import the object's 3D mesh or point cloud into your CAD program. Use tools like Mesh Enabler in AutoCAD to convert the mesh into a solid. Draw over the top of the 3D scan to recreate the part. Recreating imported 3D scans helps you better understand mesh topology while building your modeling skills. Measure Dimensions Taking accurate physical measurements of an object and replicating it in CAD is a reverse engineering fundamental. To practice: Use calipers, micrometers, measuring tapes to capture dimensions. Sketch the object from different views. Model the part in CAD based on the measurements. Practicing this process will improve your skills in technical sketching, visualization, and attention to detail. Recreate from Photos With only reference photos, carefully model the object in CAD. This teaches you to: Infer 3D geometry from 2D photos. Estimate proportions and measurements. Pay attention to perspective distortion. Recreating objects from images exercises your eye for detail. Doing projects like these builds your reverse engineering muscles. Analyzing and reproducing existing objects flexes your research, critical thinking, and modeling skills. Master reverse engineering, and you can model just about anything. Sculpture and Art One of the best ways to enhance your CAD skills is by using the tools for creative purposes. With the ability to model any shape in 3D, render photorealistic materials, and animate designs, CAD software lends itself well to digital sculpture and art. Whether you want to create abstract geometrical shapes, natural forms inspired by nature, or any object imaginable, using CAD for art provides endless possibilities. The precise modeling capabilities allow you to design intricate objects full of fine detail. And utilizing functions like lofting, extruding, sweeping, and surfacing enables you to craft smooth, organic 3D forms. When sculpting in CAD, you have complete creative control over the materials and textures. Apply glossy or matte finishes, reflective properties, transparency, bump mapping, displacement, and more to generate photorealistic surfaces. Experiment with metal, plastic, glass, wood, and custom materials. Rendered artwork made in CAD can look indistinguishable from real physical objects. Some ideas for artistic projects include: Create abstract geometric sculptures with patterns of holes, ribbing, curves and other interesting shapes. Model smooth natural forms like trees, plants, seashells or the human body. Study reference images for an organic look. Design surreal, imaginative objects by combining elements from multiple real-world sources. Make artistic visualizations of architecture, vehicles, furniture or other CAD models by rendering them artistically. The freedom of digital sculpting in CAD allows you to make anything imaginable. Let your creativity run wild, and use the advanced tools to add details and realism. Making art is both enjoyable and helps build CAD skills from imagining designs to modeling, texturing and rendering. Collaboration Exercises Advances in CAD software technology increasingly allow for streamlined collaboration between team members. To gain proficiency in collaborating on CAD projects, try these exercises: Practice Workflow Sharing Set up a shared project folder and invite other team members. Coordinate working on the same CAD model and track changes. Some exercises to try: Assign different building levels or components to each team member when designing a structure. Then integrate the work into a master model. Have multiple designers work on designing, rendering, and documenting a product prototype. Combine the work into a final set of drawings. Let team members add structural components like trusses and HVAC to an architectural model. Attempt to resolve any clashes and integrate the systems. Manage Versions Rather than emailing files back and forth, take advantage of built-in version control in CAD software. Set up a central file location and check-in/check-out or save iterations to review progress: Save versions at project milestones to archive progress. This also provides backup points. Assign version numbers to CAD models to denote progress and changes. Lock parts of a model to prevent unintended changes by collaborators. Review previous versions to understand when and why changes occurred. Track Changes Use built-in change tracking to record edits by each collaborator directly in the CAD model: Turn on change tracking before sharing a file to record individual contributions. Assign colors to each collaborator to visualize who changed what. Generate reports detailing the types of changes and when they were made. Accept/reject changes and clean up before final file export. Practicing strong collaboration workflows will build an important skillset for working efficiently in teams. The time investment will pay off with smoother project hand-offs and centralized tracking. Animation and Simulation Animation and simulation features in CAD allow you to bring your designs to life. Being able to animate mechanisms and simulate forces will take your CAD skills to the next level. Animate Mechanism Motion Use assembly constraints and motion studies in your CAD software to animate parts and components. See how linkages, cams, gears, and other mechanisms work through the full range of motion. Adjust components to improve the motion and check for interference. Simulate Forces Apply loads, forces, pressure, gravity, and friction to your CAD model. Run stress, strain, deformation, vibration, and thermal analysis simulations. See how your design reacts under real-world conditions. Make adjustments to improve performance. Analyze and Optimize Use integrated FEA (finite element analysis) tools to run structural, thermal, computational fluid dynamics, and other simulations. Identify high stress areas, heat build-up, fluid flow issues, and other problems. Refine your design to make it as efficient and effective as possible. Animation and simulation take your static CAD models and elevate them to the next level. Being able to test your designs digitally saves time and money compared to physical prototypes. Increase your employability and value as a CAD professional by mastering these important skills. Resources for Practice CAD software comes with tutorials and sample files, but here are some additional free resources for practice exercises and improving your skills: Free CAD Models and Drawings [GrabCAD](https://grabcad.com/) - Large library of free CAD models and drawings contributed by the community. Great for reverse engineering practice. [TraceParts](https://www.traceparts.com/) - Database of hundreds of thousands of free CAD models and product catalogs. [3D ContentCentral](https://www.3dcontentcentral.com/) - Thousands of free 3D CAD models in over 100 file formats. [NASA 3D Resources](https://nasa3d.arc.nasa.gov/) - Models, textures, and images from NASA for aerospace design. [Thingiverse](https://www.thingiverse.com/) - User contributed 3D printable models and STL files. CAD Tutorials and Training Courses [LinkedIn Learning](https://www.linkedin.com/learning/) - Online CAD courses included with LinkedIn premium subscription. Covers major CAD software. [Udemy](https://www.udemy.com/) - Affordable on-demand video courses for AutoCAD, Revit, Solidworks and more. Frequent discounts available. [YouTube](https://www.youtube.com/) - Free CAD tutorials and instructional videos for all skill levels. [Skillshare](https://www.skillshare.com/) - Subscription with courses on architectural visualization, product design, and other topics. [CADAcademy](https://www.caddacademy.edu/) - Free video tutorials and exercises for learning drafting and 3D modeling. CAD Certifications [Autodesk Certified User](https://www.autodesk.com/certification/overview) - Become an expert in AutoCAD, Revit, Inventor and more. [SolidWorks Certifications](https://www.solidworks.com/sw/support/796_ENU_HTML.htm) - Certify your SolidWorks skills with CSWA and CSWP exams. [PTC Creo Certification](https://www.ptc.com/en/support/training-certification) - Prove your expertise with Creo Parametric certification testing. [Siemens Certification](https://new.siemens.com/global/en/products/software/plm-software/nx-academic/nx-certification.html) - Official proficiency testing for NX CAD software. Regular practice with online tutorials, training courses, and certification exams will improve your CAD skills over time. The free resources above provide ample opportunity to learn new techniques and build your proficiency.
- 12 Must-Have Add-ons for Enhancing CAD Productivity in Mechanical Engineering
1. Customize the CAD Interface One of the most impactful ways to optimize your CAD productivity as a mechanical engineer is to customize the interface to suit your specific needs and preferences. Most CAD software allows you to extensively tailor the toolbars, menus, shortcuts, and workspace to streamline your workflow. For example, in SolidWorks you can right-click on any toolbar and select "Customize" to add, remove, and rearrange toolbar buttons. Be sure to include your most-used commands here for quick access. You can also customize the layout of on-screen panels like the Feature Manager design tree. Take time to browse through all menu items and toolbar options to see what's available. Chances are there are many specialized tools and options you haven't discovered yet. Add the most relevant ones to your toolbar for convenience. You can also set keyboard shortcuts and hotkeys to activate any menu item with a simple key command. Assign shortcuts to the tasks you use constantly to minimize clicks and mouse movement. Don't forget to customize your template files as well with your preferred interface setup. This allows you to start each new CAD model with your optimized environment already in place. Spending time upfront to customize your CAD software will pay off tremendously in the long run by allowing you to model faster and minimize wasted clicks and motions. Set up an interface that works for your specific role, industry, and modeling techniques. 2. Use Templates and Design Standards One of the most effective ways to boost CAD productivity is by creating templates and design standards. Templates allow you to automate repetitive design tasks by saving commonly used parts, features, annotations, and settings that you can quickly insert into new models. Some best practices for templates include: Create templates for standard parts like fasteners, bearings, brackets, etc. This avoids having to model the same components every time. Make templates for commonly used features such as holes, fillets, chamfers, text, and so on. Apply them with a single click rather than rebuilding each time. Set up templates with your desired model units, material properties, visual styles, and annotation settings. This will maintain consistency across all your designs. Save assembly templates with predefined coordinate systems, component placements, mates, and motion constraints. In addition to templates, establishing company or industry-wide design standards ensures consistency and efficiency when collaborating across teams. Some key areas to standardize include: Part naming conventions - Follow a consistent numbering or naming system for all parts and files. Annotation styles - Define text fonts, sizes, decimal precision, dimension styles, etc. to be used. Layer structure - Create standard layers for parts, sketches, axes, notes, etc. Material specifications - Set approved material choices and default properties to use. Model organization - Determine logical ways to group and structure components in assemblies. By taking the time to create robust templates and design standards, mechanical engineers can drastically reduce repetitive work and ensure all CAD models have a consistent high-quality look and feel. This organized foundation enables greater productivity. 3. Master Keyboard Shortcuts To maximize CAD productivity, you must utilize keyboard shortcuts rather than relying solely on mouse clicks. Learning hotkeys for common commands allows you to keep your hands on the keyboard without needing to grab the mouse. This helps you maintain focus while working faster. Most CAD programs include hundreds of keyboard shortcuts, which may seem daunting at first. Start by learning the shortcuts for commands you use the most. For example, zooming, panning, extruding, and constraining are tasks you'll do constantly. Refer to the shortcut references in your CAD software and print out a cheat sheet. Having a reference nearby makes it easier to learn hotkeys gradually. Also, take advantage of built-in tools like shortcut customization and macro recording to further streamline repetitive tasks. With enough practice, your hands will memorize the keyboard shortcuts. This motor memory is invaluable — you'll gain speed as frequently used hotkey sequences become second nature. Mastering keyboard shortcuts is one of the most impactful ways to enhance efficiency for any CAD user at any experience level. 4. Use Macros and Scripts Take your CAD automation to the next level with macros and scripts that can speed up repetitive design tasks. Most CAD programs allow you to record macros, then play them back with the click of a button. For example, you can record a macro that applies commonly used dimension styles, mating connectors, or appearance materials. This eliminates the need to manually apply these over and over again. For even more advanced automation, learn to write simple scripts using Visual Basic for Applications (VBA). With some basic coding knowledge, you can script complex workflows like generating a bill of materials, creating drawing templates, or running optimization studies. The time invested in learning VBA basics will pay off tremendously when applied to automating CAD. When recording macros or writing scripts, focus on automating repetitive, multi-step processes that you perform frequently. Good candidates for automation include applying materials, dimension styles, assembly mates, or generating drawing views. Start by recording macros manually, then open the code to understand it. Finally, enhance the code by adding loops, variables, conditional statements and other programming logic. With macros and scripts, you can build your own library of tools tailored to your specific workflows. Continuously look for opportunities to eliminate repetitive CAD tasks through custom automation. The efficiency gains will make you far more productive. 5. Simplify and Reuse 3D Geometry Creating efficient and optimized 3D geometry is key for enhancing productivity in CAD. Start by simplifying existing parts - remove any redundant features, consolidate multiple bodies into one, and reduce the number of sketches and extrusions needed. Complex models with intricate details may look impressive, but often lead to larger file sizes and longer computation times. Next, build a library of basic reusable parts to use across your designs. For example, make a standard set of fasteners, bearings, structural framing elements, electronic components, and other commonly used parts. Set them up with shared design properties so updates propagate across all instances. This avoids having to model repetitive content over and over. When creating new parts, design them parametrically with variables controlling the major dimensions. This makes scaling and configuring parts for different requirements much easier compared to static models. Consider end-use requirements like 3D printing tolerances, injection molding draft angles, or machining fixtures early in the design process. Optimize your models for manufacturability from the start for a more streamlined handoff later on. Following these strategies will dramatically shrink the time required to develop new designs while also improving quality. Removing unnecessary model clutter will also help reduce file size bloat over time. Taking advantage of reusable parameterized models gives you a strong foundation to build upon for all your mechanical engineering projects. 6. Utilize Parameters and Constraints Parameters and constraints allow you to drive your CAD models using variables and relationships instead of fixed values. This makes your designs more flexible, adaptable, and efficient to modify. For example, you can create global parameters that control the overall dimensions of a design. If you need to resize the entire model, you only have to change the parameter value in one place, instead of individually editing every feature and dimension. Using geometric constraints to define mathematical relationships between sketch entities is another powerful way to add intelligence to your CAD models. You can constrain a hole to always be centered on an edge, two lines to always be parallel, or the radius of a fillet to equal a specific parameter value. When parameters and constraints are used effectively, you can quickly explore design variations by changing key variable values. The model will update and conform to the rules and relationships defined between its driving dimensions and geometry. Benefits of utilizing parameters and constraints include: - Ability to quickly edit and update designs by changing variable values - More efficient design iteration by defining intelligent relationships - Flexibility to create design variants from the same model - Automation of design tasks that were previously manual and repetitive - Reduction in errors caused by inconsistent or arbitrary modeling decisions - Easier collaboration by defining design intent through variables and rules With some initial investment to set them up properly, parameters and constraints will enable you to work smarter and faster throughout the CAD modeling process. They help capture engineering design intent and make your CAD models behave more like true parametric engineering models rather than dumb geometry. 7. Manage Layers and Display States As you build complex CAD assemblies with many parts, managing layers and display states becomes critical for maintaining an organized file. Here are some tips: Organize parts onto different layers such as "Body", "Moving Parts", "Fasteners", etc. This allows you to turn visibility on/off for related part groups. Create display states like "Detailing", "Manufacturing", "Assembly" that show only relevant layers. Switch between display states to highlight what you need. Lock layers before sending files to suppliers or colleagues to prevent accidental edits. Use layer filters when generating drawings from 3D models. Display only layers needed for dimensioned views. Control layer visibility and display states in assemblies. Isolate layers to see how parts fit and function together. Create a layer structure template to start new projects with a consistent layering setup. Modify as needed per project. Proper layer management will make your CAD models easier to navigate and share with others. Invest time upfront organizing parts onto logical layers and creating robust display states tailored to different workflows. 8. Use Collaboration Tools Collaboration is key for effective CAD design in mechanical engineering. Modern CAD software includes built-in tools to enable seamless collaboration between team members and stakeholders. One essential feature is multi-user editing, which allows multiple users to work on the same CAD model simultaneously. This avoids version control issues from emailed copies and reduces delays waiting for others to complete their work. Leading CAD tools like Onshape, Fusion 360, and SolidWorks all support real-time cloud collaboration. Integrating your CAD platform with a product lifecycle management (PLM) system also enhances collaboration. Centralized data management ensures all team members access the latest models and documents. PLM also facilitates approvals, change orders, bill of materials, and more. Finally, collaboration requires easy file sharing. Most CAD tools allow exporting to universal neutral formats like STEP, SAT or Parasolid for non-CAD users. Cloud storage integrates directly in many CAD apps for seamless sharing. For external partners, secure file sharing portals also enable controlled access. With real-time multi-user editing, PLM integration, and flexible file sharing, mechanical engineers can work together across teams and locations. Smooth collaboration ultimately results in higher quality designs produced faster. Integrate Simulation and Generative Design By integrating CAD with simulation and generative design tools, mechanical engineers can rapidly test digital prototypes and explore innovative design options guided by artificial intelligence. Performing simulation directly within your CAD environment eliminates the need to export geometry and import it into a separate simulation software. This streamlined process allows you to quickly evaluate how a design will perform under real-world conditions. Some key analyses to run using CAD integrated simulation include: Structural analysis to validate strength and durability Thermal analysis for heat flow and operating temperatures Fluid flow analysis for fluid dynamics and aerodynamics Motion analysis to simulate mechanisms and moving parts Generative design powered by artificial intelligence takes exploration to the next level. By defining design goals and constraints, engineers can generate a wide range of design concepts optimized to meet performance criteria. The AI explores design spaces human engineers may never conceive of, leading to groundbreaking innovations. By incorporating simulation and generative design into the early stages of the design process, engineers can rapidly test and refine digital prototypes before spending time and money building physical prototypes. This fail fast, fail early approach powered by CAD productivity add-ons leads to better designs produced faster at lower cost. 10. Continuously Learn and Optimize Staying up-to-date with the latest CAD techniques and features is crucial for boosting productivity as a mechanical engineer. Here are some tips: Attend CAD training courses and webinars - Formal training is the fastest way to pick up new skills and learn how to use all of the features your CAD software offers. Look for courses offered by your software provider or third parties to level up your knowledge. Read CAD blogs and discussion forums - The CAD community is an incredible source of knowledge. Reading engineering blogs and participating in forums is an easy way to pick up new tips and tricks. Follow experts in the field and stay on top of emerging best practices. Experiment and play around in CAD - Dedicate time to just try out different tools and capabilities in your CAD system. Seeing how things work first-hand is the best teacher. Explore menus and settings to uncover new ways to enhance your workflow. Learn keyboard shortcuts - The more shortcuts you know, the faster you can work. Make an effort to memorize new shortcuts and hotkeys to bypass repetitive menu clicks. Watch video tutorials - A wealth of free video tutorials and courses can be found online. Watching over-the-shoulder videos is a great way to quickly absorb new techniques. Optimize your hardware setup - Using multiple monitors, a 3D mouse, and custom macro keyboards can improve efficiency. Invest in ergonomic hardware tailored to CAD work. Clean up files and settings - Take time periodically to purge unused files, clean up custom settings, and optimize your interface. A decluttered CAD environment promotes productivity. Ask colleagues for tips - Leverage the expertise of your team members. Ask questions and find out what tricks others are using to get work done faster. Continuously improving your CAD skills and optimizing your workflow will allow you to get more done in less time as a mechanical engineer.
- 20 Advanced Techniques to Improve Your Mechanical CAD Skills
Learn Keyboard Shortcuts Keyboard shortcuts are essential for increasing efficiency and productivity when using CAD software. CAD programs like AutoCAD, Solid works, CATIA, and more utilize shortcuts to execute commands quickly without clicking through menus and toolbars with the mouse. Mastering keyboard shortcuts can shave significant time off mechanical design and drafting workflows. The main CAD shortcuts involve commands for selections, drawing tools, modifications, layers/views, dimensioning, and utilities. For example, shortcuts like "E" for Erase, "F" for Fillet, and "M" for Move are used constantly in AutoCAD. Solid works uses "W" for Sketch, "A" for Arc, and "Ctrl+D" for Dimension. CATIA relies heavily on the F1-F12 function keys for major commands. Learning shortcuts speeds up repetitive CAD tasks immensely. Designers can activate commands in 1-2 keystrokes versus clicking through menus and toolbar icons. This adds up to major time savings over the course of a project. Shortcuts also keep hands on the keyboard, improving design flow and reducing strain from constant mouse movements. Tips for learning CAD shortcuts include printing a shortcuts cheat sheet for quick reference, using sticky notes on your monitor as reminders, and practicing shortcuts while completing CAD tasks until they become muscle memory. It's better to start with the 10-20 most useful shortcuts and gradually add more over time. Also, focus on shortcuts relevant to your CAD program and industry rather than trying to memorize them all. Consistent practice is key for training your hands and brain to use shortcuts automatically. Master the Fundamentals Before jumping into advanced CAD techniques, it's critical to build a strong foundation in the fundamentals. Mastering the basics allows you to work more quickly and accurately as you progress to more complex projects. Understand Geometry Geometry is the mathematical foundation behind every CAD design. Having a solid grasp of geometry principles such as planes, surfaces, shapes, dimensions, and spatial relationships will make you incredibly effective with CAD software. Take time to learn geometric concepts like sketching, extruding 3D shapes from 2D profiles, boolean operations like addition/subtraction, and geometric constraints. Master Math and Measurements Since CAD software deals with precision down to fractions of millimeters, having excellent math skills is a huge asset. Brush up on trigonometry, geometry, algebra, and your ability to work with all types of measurements and convert between units. Being able to model parts and assemblies to exact, real-world specifications gives your CAD work professional accuracy. Learn the Interface and Basics First Every minute spent learning the basics thoroughly will save you hours down the road. Before jumping into modeling, master fundamental functions like: Navigating in 2D and 3D space Creating and modifying geometry with sketching tools Adding constraints and dimensions Extruding, revolving, sweeping, lofting Applying materials and appearances Generating technical drawings from 3D models Building skills systematically from the ground up will accelerate your CAD learning curve and prepare you for more advanced techniques. Be patient, and focus on really mastering the basics. Practice Regularly To truly master CAD software and improve your skills, you need consistent, daily practice. The more you use CAD programs, the more proficient and efficient you will become. Set aside time each day to work in CAD, even if you don't have an active project. Practice modeling objects, drafting detailed drawings, using different tools and commands, and going through all the steps of the design process. Don't just practice the basics - challenge yourself to use advanced features and tools as well. The more you explore the software, the more techniques you will learn. Make a point to try out new shortcuts, commands, and features. Read tutorials and watch videos to find tips and tricks you haven't used before. Experiment with add-ons and plugins to expand your capabilities. Step outside your comfort zone to gain proficiency. Pay attention to your speed and efficiency as you practice. Look for ways to streamline your workflow, reuse design elements, and avoid repetitive actions. The more you practice intelligent techniques, the faster your daily CAD work will become. Time yourself to benchmark your improvements. Daily practice is essential for developing true mastery of CAD software. Consistently challenge yourself to use new features, improve your speed, and hone your skills. Regular practice makes perfect. Use Efficient Hardware Mechanical CAD software requires robust computing power to function smoothly and quickly. Investing in high-performance hardware will significantly improve your CAD experience. Many CAD operations like rendering complex 3D models and assemblies can tax your system resources. Ensuring you have sufficient RAM, a fast processor, and a powerful dedicated graphics card is essential. When shopping for a CAD workstation or upgrading your current system, focus on these key components: Processor: Look for an Intel Core i7/i9 or AMD Ryzen 7/9 CPU with at least 6 physical cores. More cores will allow you to multitask and quickly switch between CAD tools. RAM: 16GB is the minimum, but 32GB or more is recommended for complex 3D modeling. This allows you to work with large assemblies without slowdowns. Graphics Card: An NVIDIA Quadro or AMD Radeon Pro card with at least 4GB of memory is ideal. These are optimized for CAD versus gaming cards. Storage: Opt for a fast SSD (500GB minimum) for your operating system and programs. Then add a larger HDD (1TB or more) for files. Don't neglect your other hardware either. A high-resolution monitor allows you to see details clearly. And using a mouse or pen designed for CAD makes modeling more comfortable and precise. While building a new system may seem costly upfront, it will save you time every day compared to struggling with inadequate computing power. And it enables you to work faster and handle more complex CAD projects overall. Learn 3D Modeling Once you have mastered 2D drafting in CAD software, the next step is to move into 3D modeling. The ability to create 3D models opens up many more possibilities for your designs. Here are some key aspects of 3D modeling: Moving from 2D to 3D The mindshift from 2D drafting to 3D modeling requires thinking in three dimensions. You need spatial reasoning skills to manipulate objects in 3D space. With 3D CAD models, you can fully realize your design intent. You can view the model from any angle and ensure form, fit, and function. Start simple when moving to 3D. Begin by extruding 2D drawings into simple 3D shapes. Slowly increase complexity as your skills improve. Tools for 3D Modeling Common tools for 3D modeling include extrude, revolve, loft, sweep, fillet, and chamfer. Master these tools to efficiently build 3D geometry. Booleans like union, subtract, and intersect allow you to combine 3D shapes in creative ways. This helps develop complex forms. Rendering tools create photorealistic images and animations. This is useful for visualizing designs. Applications of 3D 3D modeling is used for engineering of mechanical parts and assemblies, architectural designs, medical devices, and more. With additive manufacturing like 3D printing, 3D models can be fabricated directly from CAD files. This facilitates rapid prototyping. Digital mockups of designs with realistic visuals and motion studies can be generated from 3D CAD data. This is used for design reviews. Data-rich 3D models enable advanced analysis like computational fluid dynamics and finite element analysis for assessing product performance. Simplify Geometry Simplifying geometry in CAD can lead to huge improvements in performance and productivity. By reducing the number of vertices, edges, and small details, you allow CAD software to process designs faster. This enables you to iterate quicker and speeds up critical tasks like rendering and analysis. Here are some tips for simplifying geometry: Eliminate any small fillets, holes, pockets, etc that aren't critical. Small features are computationally expensive. Reduce the number of vertices used to define curves and surfaces. Use larger, smoothly flowing radii instead of many small segments. Convert 3D meshes to solid models when possible - they have far fewer calculations. Use revolved, extruded, and swept features instead of drawing complex 3D geometry from scratch. Delete duplicate entities and combine multiple bodies into one when practical. Avoid over-defining sketches. Use dimensional constraints wisely. Cull back areas of a model the user won't see. Leverage model symmetry to build only one half. Use simplified representation for standard components like fasteners, welds, and bearings. Save highly detailed versions incrementally in case you need to revert. Simplifying designs takes experience but can speed up your workflow tremendously. It enables you to spend time on important design decisions rather than waiting for your CAD system to catch up! Specialize to Stand Out Specializing in a particular area of mechanical CAD can help you develop deep expertise and stand out from other designers. Consider focusing your skills in one of these key areas: Architecture Architectural CAD skills are in high demand for designing buildings and interiors in 2D and 3D. Master architectural visualization techniques to create stunning designs. Develop proficiency in software like Revit, AutoCAD Architecture, and SketchUp. Understand building codes and standards. Engineering Mechanical engineering CAD builds skills in designing industrial machinery, manufacturing tools, components, and more. Key skills include parametric modeling, assembly modeling, and simulation analysis. Master CAD software like SolidWorks, CATIA, and Inventor. Product Design Consumer product designers use CAD for everything from electronics to housewares. Develop skills in industrial design aesthetics, ergonomics, and manufacturing. Get experience with surfacing, organic shaping, and rendering photorealistic images. By specializing, you can provide unique value as a CAD designer and be seen as an expert. Find an area that fits your passions and interests. Attend specialized training programs and earn niche certifications. Build a portfolio of work focused within your chosen specialty. Join industry organizations and networks to build your reputation. Collaborate and Learn From Others Collaborating with other CAD designers and engineers is a great way to improve your skills and get constructive feedback. Here are some tips: Join online CAD communities - There are many online forums and groups dedicated to CAD where you can connect with other users, ask questions, and share knowledge. Being an active part of the CAD community will expose you to new techniques and perspectives. Attend local CAD meetups - Look on sites like Meetup.com for CAD user groups in your area that hold regular in-person meetings. This is a great way to meet other local CAD professionals across different industries. Find a mentor - Having an experienced CAD designer review your work and give feedback can be invaluable. A mentor can point out areas for improvement and share industry best practices. Reach out through your network or sites like LinkedIn. Participate in team projects - Collaborating on CAD projects exposes you to different design approaches and build teamwork skills. Working in a team lets you play to your strengths while learning from others. Give and receive feedback - Don't be afraid to share your CAD work on forums and ask for constructive feedback. Offer helpful feedback on others' designs as well. Listening to different perspectives will strengthen your skills. Learn from critiques - Develop thick skin and don't take negative feedback personally. Critiques ultimately make your CAD work better. Focus on understanding why something doesn't work and how you can improve. Collaborating and learning from CAD professionals, even informally online, can dramatically improve your skills and design capabilities over going it alone. Being open and humble will help you master CAD faster. Stay Updated on Technology Staying updated with the latest CAD technology is critical for improving your skills and producing innovative mechanical designs. There are several key areas to focus on: New Software As new versions of CAD software are released, take time to learn about new features, tools, and capabilities. Autodesk and other vendors frequently add advanced modeling, collaboration, and automation features that can make you more productive. Attend webinars, read release notes, and experiment with new versions to maximize your skills. Emerging Technologies Keep an eye on emerging technologies like virtual reality (VR), augmented reality (AR), and generative design. As these technologies mature, they will likely integrate with CAD software and open up new design approaches. Get hands-on experience with new tech when possible. Hardware Upgrades CAD software demands powerful hardware. Monitor hardware advances in areas like GPUs, CPUs, displays, input devices, and cloud computing. Upgrade your system when feasible to take advantage of faster performance and improved capabilities. But don't upgrade too frequently, as CAD software lags behind new hardware. Staying abreast of the latest CAD technologies will help sharpen your skills, inspire creativity, and position you for success as a mechanical CAD designer. Make learning new tech a lifelong habit. Develop Soft Skills CAD designers need more than just technical prowess - soft skills are equally important for success. Developing strengths in communication, problem-solving, time management, and creativity will make you a well-rounded CAD professional. Communication Having strong communication skills enables you to clearly convey your design intent and collaborate effectively with colleagues, clients, and team members. Practice explaining your design decisions, articulating technical specifications, describing manufacturing considerations, and more. The better you communicate, the better your designs will turn out. Problem-Solving CAD design involves constantly solving problems and improving workflows. Sharp analytical thinking and problem-solving skills let you quickly diagnose issues, devise solutions, and overcome obstacles. Always approach problems with a solution-oriented mindset. Consider all options and think creatively. Time Management Juggling multiple design projects and competing priorities takes planning and organization. Time management skills allow you to budget time wisely, meet tight deadlines, and avoid wasting time while modeling. Use productivity tools to stay on track. Prioritize urgent tasks without neglecting larger goals. Creativity While technical aptitude is crucial, creative thinking sets great designers apart. Allow time for free-flowing creative concepting instead of jumping straight into modeling. Sketch ideas by hand to stimulate visual thinking. Look at problems from different angles. CAD tools bring your creativity to life, so focus first on nurturing an inventive mindset.
- 7 Common Mistakes to Avoid in Mechanical CAD Design
Not Backing Up Your Work Failing to properly back up your CAD work can lead to countless lost hours and missed deadlines if files become corrupted or lost. Make it a habit to frequently save your CAD work-in-progress to avoid losing progress should software crash or hardware fail. Set reminders to save your work every 15-30 minutes. Additionally, be sure to back up your CAD files regularly to both local and cloud-based external drives. Perform daily or weekly backups to create restore points you can roll back to if needed. Store backups in more than one location, like an external HDD and a cloud drive. This ensures you have multiple copies if one backup fails or gets corrupted. Keep at least one backup copy offsite or in cloud storage. This guards against loss from local disasters like fires, floods or theft. Losing your only backup means starting CAD work from scratch, so protect yourself with an offsite cloud or external drive copy. Making regular backups takes little time but provides peace of mind your CAD data is safe. Poor Layer Organization Proper layer organization is crucial for efficient CAD design and collaboration. Unstructured layers lead to a disorganized mess that's difficult to edit and share effectively. Here are some tips for optimizing your layer workflow: Use structured layers - Organize your CAD drawing into logical layers based on function or object type. For example, create separate layers for dimensions, text, mechanical parts, electrical components, etc. This makes elements easy to locate and modify. Group related objects - Keep objects that relate to each other on the same layer. This ensures they move together if you need to make adjustments. All holes, bolts, and fasteners for a single part should be grouped. Name layers logically - Use descriptive names that indicate the purpose of each layer. "Dimensions," "Text," "Fasteners," and "Moving Parts" are clear names. Overly generic names like "Layer 1" are confusing. Limit layer numbers - Too many layers clutter the list and make specific ones harder to find. Try to consolidate and only create layers when truly needed. Establish standards - Develop a consistent layer naming convention across projects and teams. Standards help new designers orient faster. Lock finished layers - Lock reference layers like those containing a title block to prevent accidental changes. Also lock finalized product layers before sending to others. With structured CAD layers, you can easily control visibility, apply edits globally, and hand off projects more smoothly. Organized layers keep file sizes smaller and prevent a tangled mess. Flouting Standards Ignoring industry standards is one of the biggest mistakes you can make in mechanical CAD design. While it may seem easier in the moment to forge your own path, doing so will likely come back to bite you down the road. Standards exist to make collaboration, manufacturing, and maintenance smoother for everyone involved. Deviating from them can lead to miscommunication, errors, delays, and extra costs. It's not worth it! Here are three key areas where you should follow standards in your CAD work: Use Standard Annotations Get in the habit of using standard callouts, labels, and annotations in your drawings. This includes things like standard fastener callouts, surface finish symbols, weld symbols, and more. Follow guidelines like ISO or ASME standards so your annotations are clear to others. Follow Industry Best Practices Research and follow industry best practices for your specific field. For example, manufacturing companies likely have standards around items like layer naming, line weights, title blocks, etc. Follow those standards to avoid extra work later. Standardize Your Own Methods While you should follow broader standards, also make sure to standardize your own methods within a project or organization. For example, standardize things like units, dimensioning style, line types, and more. Being consistent in your own work will help avoid confusion. Sticking to mechanical drafting and design standards may require more upfront work, but it pays off hugely in avoiding errors and improving collaboration down the line. Don't cut corners here! Follow the standards. Overlooking Printability When creating CAD models, it's essential to keep printability in mind. If you don't consider the limitations of 3D printing or other fabrication methods, you may end up with a design that simply can't be manufactured. One of the key aspects of printability is checking the sizing and resolution of your CAD model. Extremely small features or thin walls may not print properly on some machines. Review the minimum size capabilities of your printer and avoid details that are below this threshold. You should also test how your CAD model looks when rendered in different views. Some geometries and surfaces may appear feasible on screen but are actually too complex to fabricate. Rotate the model and zoom in on different areas to spot potential printability issues. Finally, be sure to thoroughly review the model before sending it to print. Look for surface defects, intersections that may cause problems, and any features that seem questionable. It's better to catch printability problems while still in CAD than to waste time and material discovering them during the printing process. With careful inspection and test prints, you can feel confident your design will fabricate as intended. Unrealistic Tolerances When designing parts in CAD, it's important to specify tolerances that are actually achievable in manufacturing. Being too tight with your tolerances can lead to parts that are impossible to produce. This not only wastes time and money, but can stall the entire project. To avoid this issue, make sure you have a solid understanding of the manufacturing processes that will be used. What are the capabilities and limitations of the machines? Talk with your manufacturers early in the process to get their input. You'll also want to research common tolerances for different features, materials, and operations. Allow tolerances that are reasonable for each feature's function and manufacturability. Avoid impossible specs that sound good on paper but can't actually be held in production. Tight tolerances aren't free - they add significant cost. Make sure you have justification for each tight tolerance, or else relax it to save on manufacturing. With smart tolerance choices, you'll avoid scrapped parts and keep the project on track. Dimensioning Errors Proper dimensioning is crucial for a successful mechanical CAD design. Dimensions fully define the size, position and tolerances of every feature in a CAD model. Even a single dimensioning mistake can lead to costly errors and delays down the line. When adding dimensions in CAD, it's essential to fully define all features so there is no ambiguity. Every hole, curve, angle and surface must be precisely dimensioned. Leaving anything underspecified invites confusion. You should also double check dimensions for any potential conflicts. For example, make sure a hole doesn't get two different diameters depending on the view. Using the proper dimensioning notation is equally important. Follow accepted standards like ISO or ASME Y14.5M to ensure your dimensions are clear and readable. Mixing notation styles or using sloppy terminators will erode understanding. Stick to standard terminology for maximum clarity. By carefully dimensioning every feature and cross-checking for errors or conflicts, you can avoid headaches during manufacturing. Taking the time to add proper dimensions ultimately saves time and money compared to scrambling to fix mistakes later on. Precise, unambiguous dimensioning is one of the hallmarks of a professional CAD design. Weak Collaboration Effective collaboration is critical in CAD projects with multiple stakeholders. Without proper version control, task delegation, and communication, designs can quickly become disjointed and compromised. To enable seamless teamwork: Use version control like Git to track changes and prevent conflicts. With a VCS, multiple designers can work on the same files simultaneously without risk of overwriting work. Clearly assign tasks so responsibilities are not duplicated or neglected. Break the project into logical sections and designate owners. Hold kickoff meetings to align on goals. Communicate frequently through calls, messages, and design reviews. Sharing context and connecting often avoids dropped balls and misunderstandings. Document decisions in a central place like a wiki or README. Log why choices were made to pass on key info. Automate notifications on changes so teammates are aware of updates. No one should be surprised by merges. With deliberate coordination baked into the process, projects proceed smoothly across locations and time zones. A culture of openness and accountability keeps everyone on the same page. Limited Testing Not thoroughly testing your CAD designs can lead to costly manufacturing errors and delays down the line. It's crucial to test your digital prototypes before sending designs off for production. Here are some tips on properly testing your CAD work: Test prints and prototypes - Use your own 3D printer or a printing service to create physical models of your design. Examine test prints closely and make tweaks in CAD as needed. Test different orientations, settings, and materials. Get peer reviews - Have colleagues and experts review your CAD design to spot issues you may have missed. Fresh eyes can find flaws in dimensions, functionality, manufacturability, etc. Confirm specs are met - Compare prototypes thoroughly against product specifications. Ensure all critical dimensions, tolerances, and performance criteria are met. Refine the CAD as required. Simulate real-world conditions - Test parts under expected loads, vibration, temperatures, and other conditions. Use simulations and physical testing to confirm the design withstands real-world use. Refine and retest - Go through multiple iterations of printing, reviewing, testing and refining your CAD work. Each cycle will improve the design and catch more errors. Thorough testing takes time but avoids far greater costs down the road. Build plenty of evaluation into your design timeline, and don't finalize CAD until rigorous testing is complete. Smart testing processes will lead to robust, production-ready designs. Not Considering Materials When designing parts in CAD, it's crucial to consider the materials you plan to use for manufacturing. The material choice affects the part's strength, weight, manufacturability, durability, and cost. Selecting an inappropriate material can lead to failure down the line. For example, choosing a brittle plastic for a flexible component that undergoes repeated bending may cause it to crack or snap under stress. Or using a heavy metal alloy for a component intended to be lightweight can make the end product too heavy. To avoid problems, research the properties and capabilities of candidate materials early in the design process. Factors to consider include: Strength - How much force can the material withstand without permanent deformation or fracture? Hardness - What type of wear will the material be subjected to? Softer materials may scratch or erode. Corrosion resistance - Will the operating environment degrade or oxidize the material? Thermal properties - How does the material perform at temperature extremes? Will it soften, melt, or become brittle? Weight - What density range is acceptable for the application? Lightweight materials can reduce shipping costs. Aesthetics - Surface finish, color capabilities, and visual appeal may be important. Cost - Expensive materials like titanium may be justified for high-performance applications. Manufacturability - Is the material easy to fabricate using available methods? Complex shapes in hard metals increase machining time and cost. Sustainability - Can the material be sourced responsibly and recycled after use? Also analyze how different materials impact other aspects of the design like part consolidation, fastening, and assembly. The optimal material minimizes waste while meeting functional requirements. Thoroughly research prospects before finalizing your choice. Getting this fundamental decision right early on will prevent headaches later. Rushing the Process One of the most common mistakes in CAD design is trying to move too quickly through the process without proper planning. It's tempting to jump right into modeling when excitement and inspiration strike. However, adequate time must be devoted to the design process to achieve high-quality results. Rushing leads to frustration when setbacks occur or details are overlooked. To avoid problems, invest sufficient time upfront to develop realistic timelines. Be aware that the modeling stage often takes longer than expected. Build in review stages where experienced designers and engineers can provide feedback on the CAD model. Plan for multiple rounds of revisions and refinements to work out any issues. Trying to fast-track a design may seem efficient initially, but rarely ends well. Projects without cushion for unexpected developments or modifications usually require extensive rework later. Allow ample time for each step, from initial concept sketches to final documentation. While passion is important, patience is equally crucial when bringing a design to life in CAD.
- 15 Tips for Efficient 3D Modeling in Mechanical Engineering Projects
Learn the Basics of Your 3D Modeling Software Choosing the right 3D modeling software is crucial for mechanical engineering projects. The software needs to have robust CAD and simulation capabilities suited for mechanical design. Some of the most popular options used in the industry include: SolidWorks - One of the most widely used 3D CAD programs for mechanical design. It provides powerful modeling, drafting, simulation, product data management, and documentation tools. SolidWorks is known for its intuitive user interface and productivity features. AutoCAD - A general CAD software with strong 2D drafting and 3D modeling capabilities. AutoCAD is highly customizable and supports mechanical engineering workflows through specialized extensions. It offers tools for design visualization, electrical design, and product simulation. PTC Creo - Formerly known as Pro/ENGINEER, Creo enables 3D CAD modeling with options for photorealistic rendering and CAE/CAM support. It offers reverse engineering capabilities and tools to simplify complex designs. Creo is popular in automotive, aerospace, machinery, and high-tech industries. On shape - A newer cloud-based CAD solution that runs on any browser. It allows easy collaboration and micro version control on designs. Onshape provides professional features like large assembly design, production-level surfacing, and robust simulation tools. Once you select the right 3D modeling software for your needs, invest time in thoroughly learning it. Go through tutorials, videos, and the software help/documentation to become familiar with the interface, capabilities, and workflows. Master the fundamentals like sketching, extruding, constraining, and dimensioning objects before moving to more complex modeling. Developing proficiency early on will speed up the 3D modeling process tremendously. Follow Design and Modeling Guidelines Before starting a 3D modeling project, it's crucial to review the design and modeling guidelines for your specific industry and application. Having clear standards in place will ensure your models meet all necessary requirements for form, fit, and function. For mechanical engineering projects, your models will likely need to integrate with manufacturing processes, testing simulations, prototyping, and other systems. Check for any CAD modeling requirements from manufacturers or fabricators you may use. Formats like STEP, IGES, and Parasolid are common. You'll also want to follow general best practices for your 3D modeling software. For example, in SolidWorks it's recommended to: Fully define sketches to avoid issues. Use dimensions and geometric constraints to lock down sketch geometry. Limit the number of extraneous sketch entities. Keep sketches as clean and simple as possible. Minimize unnecessary features. Whether extrusions, fillets or holes, simplify the design if they don't serve a purpose. Maintain consistent modeling techniques and approaches across parts and assemblies. Follow templates if available. Additionally, set modeling tolerances appropriately for the level of accuracy and precision required in the design. Determine the units and decimal places needed. Besides technical guidelines, make sure to clarify stylistic expectations and conventions as well. This includes things like part naming schemes, organization of features and assemblies, and design element preferences. Setting modeling standards early on will optimize efficiency throughout the project. It also facilitates collaboration when multiple designers are involved. Taking the time to understand and implement modeling guidelines will lead to higher quality results. Start Simple Before Adding Complexity When creating a 3D model in mechanical engineering, it's important to start with simple shapes before adding layers of complexity. Many inexperienced 3D modelers make the mistake of jumping right into modeling every detail of a complex design. However, this often leads to frustration, wasted time, and subpar models. Instead, begin by modeling the basic overall shapes that define the core geometry of your design using primitive shapes like cubes, cylinders, cones, and spheres. Get the approximate proportions, alignments, and spatial relationships locked down first. Once you have this simple foundation built, you can then begin adding finer details one element at a time. Manipulate, combine, and build upon these basic shapes to model holes, fillets, chamfers, cuts, ribs, bosses, and any other features present in the final design. Starting with a simple shape that represents the core concept helps align all the pieces as you build up complexity. It also makes edits and adjustments much easier compared to trying to tweak a complex model. Approaching 3D modeling in this layered way improves efficiency and minimizes mistakes. Use Reference Points for Alignment One of the keys to creating an accurate 3D model is identifying clear reference points to align the model properly. Having set reference points gives you anchor positions to orient the overall model correctly before adding further details and dimensions. When modeling a mechanical part, look for obvious symmetrical points and features like holes, edges, and center lines that can act as references. Markers like the corners, midpoint, or diameter of a circular or rectangular base can provide alignment guides. For an irregularly shaped part, you may need to place temporary guide geometry in the modeling software to map the boundaries. Measure or calibrate your chosen reference points against the physical object or scanned data. Double check a few points to verify consistency. As you build up the foundational geometry of your model, align it to snap precisely to the reference points. With the core aligned, you can then start adding the finer features and details with confidence that the orientation matches the real object. Maintaining alignment as you model also avoids introducing inaccuracies or distortions that can propagate through the model and be difficult to correct later. Periodically check back with the reference points to validate that everything remains aligned as you work. Taking the time to properly position a few key reference points creates an aligned foundation for 3D modeling complex objects accurately and efficiently. This alignment discipline will improve the quality and precision of your mechanical engineering designs. Focus on Necessary Details When creating 3D models for mechanical engineering projects, it's important not to overcomplicate the model with extraneous or unnecessary details. Adding too many intricate features can inflate file sizes, slow down your modeling workflow, and potentially cause errors or defects. Start by identifying the critical details that are essential for the model's purpose. This may include specific dimensions, tolerances, surface finishes, assembly requirements, and other engineering specifications. Resist the temptation to model every last nut, bolt, and screw if the extra detail does not provide value. Evaluate each detail you want to incorporate and ask yourself: Is this detail necessary for the model to convey the required information? Will adding this delay the schedule or complicate the modeling process? Can a simpler representation achieve the same purpose? Erring on the side of simplicity often produces a cleaner and more optimized model. Remove fillets, chamfers, and other non-essential elements. Consider alternatives like depicting fasteners as simple shapes rather than detailed screw models. Suppressing unnecessary features helps reduce file size and modeling time. This allows you to focus your efforts on what matters most - creating an accurate 3D representation of the key engineering elements required in the final product. Keeping the model straightforward also reduces the likelihood of introducing defects. With a clear understanding of the model's purpose and intended use, you can zero in on the vital details that fulfill the requirements. Resist over-modeling just for the sake of complexity. The most effective mechanical engineering models focus on essential details. Validate Models from All Angles Once you've created an initial 3D model, it's crucial that you thoroughly validate it by viewing from all angles before finalizing. Many defects and errors can be missed if you only view the model from the default angle. Make sure to rotate and manipulate the model to view it from the top, bottom, sides, front, back, and any angled perspectives. Zoom in closely during the inspection to check for small defects, inconsistencies, or inaccuracies that are hard to see from a distance. Flaws in your model may include: Misaligned faces or edges Gaps, holes, or cracks Intersecting components Incorrect measurements or dimensions Features that should be symmetrical but are slightly off Carefully inspect fillets, curved surfaces, and any fine details in the model by orbiting around those areas. Subtle defects can lead to failure during simulation or problems in manufacturing, so this thorough validation process is essential. Don't rely solely on rendered views either. Check the wireframe view of your 3D model to ensure all edges and vertices align precisely. Use intersecting planes to slice your model and inspect the interior cross-sections. Your model should be watertight and printable when viewed from any orientation. Take your time on this step, as fixing defects later requires rework. Verifying accuracy from all angles now will prevent headaches down the road. Test Models Before Finalizing Before you finalize a 3D model and send it to manufacturing, it's crucial to test the model thoroughly. One of the best ways to do this is by 3D printing sample versions of your model. 3D printing allows you to create physical prototypes so you can inspect your design from all angles. It also shows how the model will actually look and function in the real world. When 3D printing test models, look for any defects, gaps, or issues with fit and finish. Check that all measurements are precise and components fit together as expected. Examine the model closely to ensure it meets the specifications and design intent. Testing a 3D printed sample can reveal flaws in the model that are hard to spot on screen. For example, you may find certain features are too thin or delicate in the physical model. Or sections that should connect smoothly end up misaligned when printed. Making adjustments to the 3D model and printing additional test samples lets you refine the design until the model prints accurately. Don't finalize a 3D model for manufacturing until you're fully satisfied with the 3D printed test version. This hands-on evaluation step helps validate your design and avoids discovering issues after production has already begun. Taking the time to iterate and perfect 3D printed models leads to higher quality final products. Optimize File Size to Improve Performance When creating 3D models for mechanical engineering projects, file size can become a major constraint. Complex models with intricate details usually result in very large files. This causes several issues: Slows down computers when opening and manipulating the files. The larger the file, the more RAM and processing power needed to work with it smoothly. Difficult to share and transfer the files. Emails and cloud services often have limits on attachment sizes. USB drives may not have enough free space. Bottlenecks for 3D printing. The printer will take longer to process and slice a large file in preparation for printing. There are a few techniques you can use to optimize your 3D model file size without sacrificing quality: Start with the simplest base geometry that conveys the overall shape. Avoid adding tiny details too early. Use chamfers and fillets sparingly. Every curved surface adds to file size. Replace detailed or curved surfaces with angular faces when possible. Limit the number of unique custom features. Reuse patterns instead of reinventing the wheel. Remove any hidden or internal geometry that isn't essential. Check the polygon count. Reducing overly complex meshes will drastically reduce file size. Export using binary formats like .step instead of text-based .igs or .stl. By simplifying the geometry and removing unnecessary complexity, you can shrink 3D model files significantly. The model may look identical visually, but the file size improvements will optimize performance. Communicate with Manufacturers Effective communication with manufacturers is critical to ensure your 3D models meet requirements and can be accurately produced. Here are some best practices: Provide the manufacturer with all necessary views - front, back, sides, top, bottom, isometric, etc. This allows them to fully visualize the part from all angles. Include precise measurements, tolerances, and material specifications in notes attached to the model or on a separate drawing. Any critical dimensions or callouts should be clearly labeled. Make yourself available to answer any questions the manufacturer may have after reviewing the 3D model and drawings. Be responsive to requests for clarification or additional details. For complex parts, consider creating a design checklist or manufacturing notes document. Use this to highlight key features, call out potential trouble spots, and provide guidance to optimize machining. If possible, discuss the project with the manufacturer early in the design phase. Their expertise can help identify design changes to improve manufacturability. Review material options compatible with the manufacturing method. This ensures your selected material can be feasibly machined or processed. For critical applications, request prototyping of a sample part to verify your 3D model results in an acceptable finished product before full production. Clear communication and coordination with manufacturing partners ensures your 3D model data results in quality finished parts produced correctly the first time. Refine and Iterate Models While your initial 3D models may seem complete, it's important to continue refining and iterating them based on testing and feedback. Even experienced mechanical engineers rarely get a 3D model perfect on the first try. Once you've created an initial 3D model, thoroughly test it to identify any flaws or areas for improvement. 3D print the model for physical validation. Check measurements, clearances, strength, and other parameters. Have colleagues review the model and provide feedback. Use the results of testing to make adjustments and tweaks to the 3D model. Fix any issues that are identified. Refine tolerances and clearances. Modify the design to improve strength or manufacturability. With each new version, repeat the testing process. 3D print updated models and measure the results. Request feedback from reviewers. Continue iterating in this loop until satisfied with the final 3D model. The back and forth of testing, obtaining feedback, and refining is key to developing accurate, optimized 3D models. It takes diligence, but each round of revisions brings you closer to an effective model suitable for your mechanical engineering needs. Don't be afraid to go through multiple iterations - the time investment will pay off through enhanced performance and quality.